TAMPON FALSE PCR TEST SENT DIRECTLY TO THE BRAIN 🧠
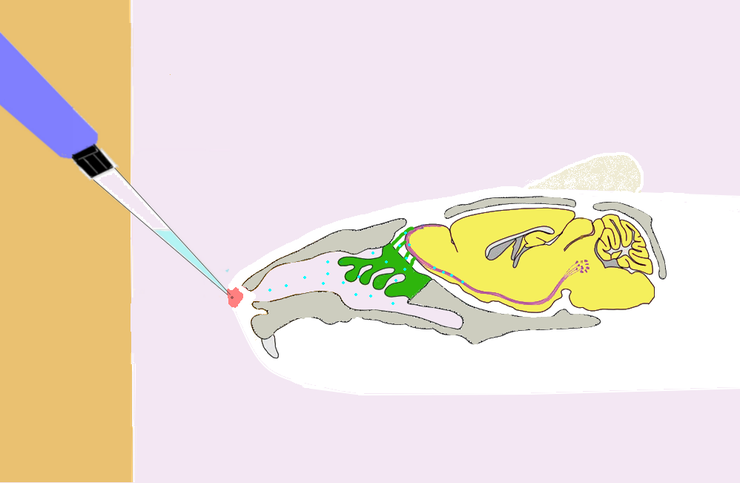
Introduction of nanoparticles into the nasal cavity of a mouse to study the transport of substances to the brain bypassing the blood-brain barrier.
Drawing in full size.
During the experiment, students 🧑 🎓 doctors found out the mechanism of penetration of nanoparticles from the nasal cavity into the brain, bypassing the blood-brain barrier (BBB). To do this, they used manganese oxide (Mn3O4) nanoparticles, which were visualized by tomography. So far, they have described several possible pathways for substances to transport substances from the nasal cavity to the brain, but the exact mechanism has not yet been determined. To see exactly how the nanoparticles enter the brain, a series of experiments were conducted to block the capture/transport of nanoparticles in neurons; the hypothesis of transport through extracellular space was also tested. The relevance of this work is the identification of ways of drug delivery and the study of the penetration of viruses into the brain bypassing the BBB.
In the human body, there is a special system of protection of the brain from the penetration of large molecules, including infectious agents - this is the blood-brain barrier (BBB). Let's take a closer look at its structure (Fig. 1). The first line of defense is the dense endothelium of capillaries connected by dense contacts. Unlike most capillaries of the body, they do not have large gaps (pores) for the passage of some plasma proteins [1]. Further on the way to the brain are pericytes (connective tissue cells) and astrocytes (auxiliary cells in the nervous tissue), which mechanically do not allow molecules larger than a certain size to pass. The blood-brain barrier does not pass substances more than 400-500 Yes by weight, depending on the properties of the substance. (By comparison, human serum albumin, the most abundant protein in the blood, has a mass of 65,000 Da.) Also, the barrier is impervious to ions, but passes fat-soluble substances, water, oxygen, carbon dioxide, some painkillers and alcohol (Fig. 1).
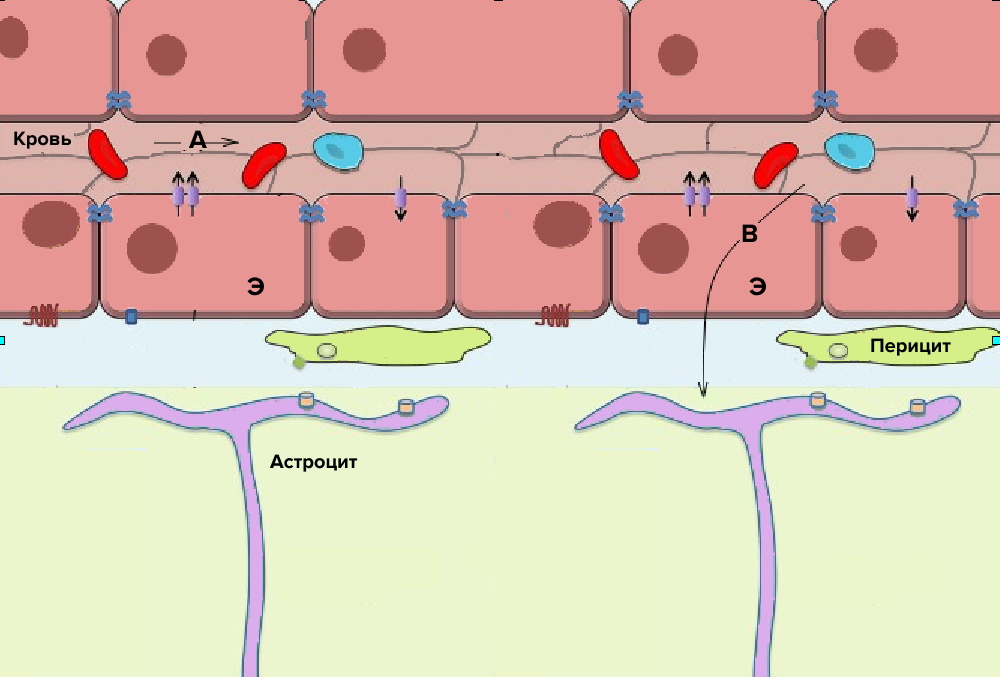
Figure 1. Diagram of the structure of the blood-brain barrier.
E - capillary cells connected by tight contacts; A - substances that do not penetrate the BBB; B - substances that penetrate the BBB in any way described below. The arrows indicate the protein systems of transport of substances in and out of the cell.
Thus, for a long time it was believed that the brain is completely protected from the penetration of certain substances from the blood, until the possibility of passage through the BBB was discovered. This method of delivery is needed primarily to deliver drugs to the nervous system, so it was important to find ways to overcome the barrier: to weaken the cellular contacts of the endothelium (cell layer) of the capillaries of the brain, to use the transport systems of substances through the capillary membrane or to penetrate the brain using endocytosis [2]. These methods have their drawbacks, for example, the destruction of dense contacts of the endothelium led to a local accumulation of substances in the brain, an increase in intracranial pressure and required considerable time to restore the barrier [3]. The use of transport systems of water-soluble metabolites to deliver the active substance to the brain imposes restrictions on the substance itself. In this case, it must either mimic the "habitual" metabolite of the cell for this transporter protein, or bind to the metabolite to pass through the membrane [4]. The transport of substances by endocytosis (capture of external material) by endothelial cells also has its drawbacks - nonspecific endocytosis is minimized in the capillaries of the brain, and specific endocytosis often involves partial transmission of the substance. For example, when iron ions are delivered by means of protein ferritin, this protein binds to a receptor on the capillary endothelium, enters the cell, releases iron ions for further transport to the brain, and then is removed from the cell back to the lumen of the capillary [5]. All of the above methods have a common nuance - the substance enters the brain through the blood, which means that the substance is distributed evenly throughout the body, so you need to take into account its systemic effect. This imposes additional restrictions and increases the time of testing a new drug. In recent decades, scientists have tried to overcome the barrier and deliver drugs using nanoparticles injected into the blood [6–8].
Nanoparticles are the collective name for a group of substances 1 to 1000 nm in size. They can have different shapes and properties, depending on the way to overcome GEB. It can be various polymers, natural or synthetic, or metal particles. However, so far, nanoparticles do not show the best results as drug transporters through the barrier if they are injected into the blood, and the way they penetrate the barrier is still a controversial issue [9]. As Francesco Eldro noted, a lot of time was spent on changing (modifying) the active substances in the composition of drugs to overcome the barrier, but much less studied the ways of their penetration into the brain [10], [11].
There are ways to penetrate the nervous system, even bypassing the stage of entering the blood. Of course, you can drill a hole in the skull and inject the substance with a needle through the barrier - this is a fairly effective way to overcome the BBB. The only drawback is that the injected substance practically does not spread through the brain [12]. But there is also a much less invasive option - to penetrate the brain through the nasal cavity bypassing the GEB. Consider why this method is of particular interest to scientists. Let us recall the structure of the olfactory system of vertebrates on the example of a mouse (Fig. 2). In the nasal cavity there is a special area of accumulation of receptor endings of neurons - the olfactory epithelium. From dendrites, the signal passes through the body, is transported along the axon and transmitted to the mitral cell, which is part of the olfactory bulb; the place of transmission is called the synapse.
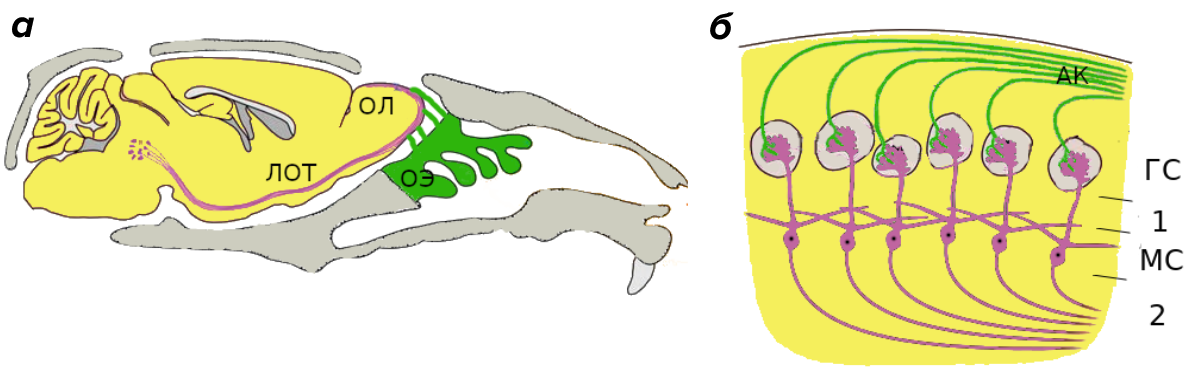
Figure 2. The structure of the olfactory system.
(a) is the structure of the olfactory system of the mouse in section. OE - olfactory epithelium, OL - olfactory bulb, LOT - lateral olfactory tract.
(b) is the construction of the olfactory bulb of a mouse. AK) - axons of olfactory receptors, HS - glomerular layer, MS - mitral layer, 1 and 2 - external and internal plexiform layers of the olfactory bulb.
The bodies of mitral cells form the mitral layer, and the axons of these cells form the lateral olfactory tract. It transmits information about smells to the centers of the brain, which process the signal. Due to the "availability" of this method of getting into the brain, which in addition does not require serious restrictions on the structure and size of substances, this topic requires a more detailed analysis.
This work is devoted to the study of the process of penetration of nanoparticles into the brain through the nasal cavity. This path is relevant not only from the point of view of drug delivery, but also from the point of view of studying the process of penetration of viruses into the brain. Everyone now hears a specific symptom of coronavirus infection - loss of smell, which indicates the possibility of non-specific penetration of any substance into our nervous system.
Experiment description and results
To more accurately visualize the transport of substances to the brain through the nasal cavity, we used manganese oxide nanoparticles (Mn3O4, ~34 nm in diameter). By inserting them into one nostril of the mouse, you can see the position of the nanoparticles during tomography (Fig. 3).
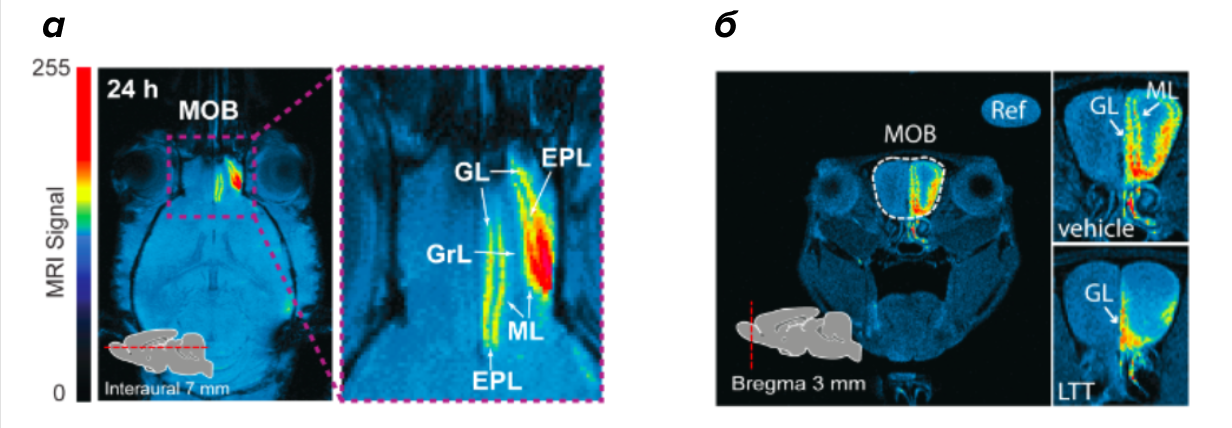
Figure 3. Tomogram of the olfactory bulb of a mouse.
(a) - the distribution of the MRI signal along the layers of the olfactory bulb (MOB): glomerular layer (GL), outer plexiform layer (EPL), mitral cell layer (ML), granular cell layer (GrL) 24 hours after the introduction of Mn3O4 nanoparticles into the right nostril. The intensity of the signal is selected using pseudo-staining (the computer paints the image in different colors depending on the intensity of the MRI signal).
(b) - a picture in the presence (LTT) and the absence (vehicle) of an inhibitor of the presynaptic activity of the neuron. White arrows show the layers: the glomerular layer (GL) and the mitral cell layer (ML).
In the course of the work, the effect of various inhibitors on the capture and transport of nanoparticles to the mouse brain was analyzed to answer the main question: nanoparticles pass from the nasal cavity through neurons or they enter the nervous system through the extracellular space [13].
To trace the path of nanoparticles from the olfactory epithelium to the olfactory bulb, specific and nonspecific endocytosis inhibitors were used, as well as substances that destroy the dense contacts of olfactory epithelial cells. The differences between endocytosis inhibitors are that specific inhibitors block the capture of a certain substance by cells, and non-specific ones reduce the overall ability of cells to endocytosis. Based on the data obtained, we concluded that the particles are absorbed by the cells of the olfactory epithelium, and do not pass between them. It can also be concluded that the capture of nanoparticles occurs without interfacing with any substance, since specific endocytosis inhibitors did not affect the level of the MRI signal.
To trace the further path of nanoparticles, we used axonal transport inhibitors (colchicine, lidocaine). Colchicine blocks the movement of loads from the body of neurons to the synapse; lidocaine inhibits the conduction of impulses in neurons due to the blocking of sodium channels (therefore, nanoparticles, once in the neuron, can not be transmitted further to the synapse). Both inhibitors significantly affected the transport of Mn3O4 nanoparticles from the nasal cavity to the brain. Thus, we showed that Mn3O4 nanoparticles penetrate the olfactory bulb through the axon, and their transport depends on the activity of the neuron.
Continuing to move into the brain with nanoparticles, we get into the outer layer of the olfactory bulb. Here, the nanoparticles are faced with a choice: either go through the synapse to the next neuron and then be transported along the lateral olfactory tract, or move through the intercellular space.
It is possible to understand this through the influence of blockers. Inhibitors of pre- and postsynaptic neuronal activity affect only the stage of transport of nanoparticles from the olfactory bulb (MOB) to the lateral olfactory tract (LOT). Moreover, only inhibitors of presynaptic activity - baclofen and LTT (levitriacetam) have a significant effect. This effect appears due to the fact that nanoparticles do not work as neurotransmitters. To induce postsynaptic activity in a neuron, the substance must bind to a receptor on the surface of the neuron and cause the appearance of an action potential in it (transmit the signal further along the nerve chain). Nanoparticles cannot bind to specific receptors; they enter the next neuron due to nonspecific endocytosis. Because of this, inhibitors of postsynaptic activity practically do not affect the transport of nanoparticles from the olfactory bulb to the lateral olfactory tract. Therefore, we can conclude: nanoparticles are transmitted transsynaptically through the brain structures responsible for the sense of smell bypassing the BBB.
Thus, on the example of magnetic nanoparticles in the experiment, it was shown that it is possible to transport particles from the nose to the brain bypassing the BBB inside neuronal cells. Moreover, the process of nasal transport of particles depends on the activity of neurons. Since these nanoparticles are similar in size and other physical properties to respiratory viruses, it can be assumed that the transport of viruses into the nervous system is possible not only by penetration through the BBB [14]. Thus, the found method of transporting nanoparticles bypassing the BBB provides the basis for the study of the transmission of viral diseases in this way, as well as for the creation of new antiviral drugs. Of particular relevance is the study of the transport of SARS-CoV-2, since one of the symptoms of coronavirus infection is the loss of taste and smell.
Conclusion
The result of the work was the confirmation of the hypothesis that nanoparticles are transported through neurons to the brain, and not through the intercellular space. We can also say that they are not only captured by olfactory receptors, but also pass through the synapse into the mitral cell of the olfactory bulb, after which they are transported through the lateral olfactory tract to the brain. Understanding the exact mechanism of transporting substances bypassing the BBB can greatly simplify the creation of drugs against known diseases - Parkinson's disease and Alzheimer's disease, and will also help expand the understanding of how viruses enter the human nervous system.
Literature
Guyton Arthur K., Hall John E. Medical physiology. Logosphere, 2008. — 857 p.;
- Yan Zhang, Donald W. Miller. (2005). Pathways for Drug Delivery to the Central Nervous System. Drug Delivery. 29-56;
- Marc-André Bellavance, Marie Blanchette, David Fortin. (2008). Recent Advances in Blood–Brain Barrier Disruption as a CNS Delivery Strategy. AAPS J. 10, 166-177;
- Yasuo Uchida, Sumio Ohtsuki, Yuki Katsukura, Chiemi Ikeda, Takashi Suzuki, et. al.. (2011). Quantitative targeted absolute proteomics of human blood-brain barrier transporters and receptors. Journal of Neurochemistry. 117, 333-345;
- Wilfred A. Jefferies, Malcolm R. Brandon, Simon V. Hunt, Alan F. Williams, Kevin C. Gatter, David Y. Mason. (1984). Transferrin receptor on endothelium of brain capillaries. Nature. 312, 162-163;
- Cláudia Saraiva, Catarina Praça, Raquel Ferreira, Tiago Santos, Lino Ferreira, Liliana Bernardino. (2016). Nanoparticle-mediated brain drug delivery: Overcoming blood–brain barrier to treat neurodegenerative diseases. Journal of Controlled Release. 235, 34-47;
- Luca Bors, Franciska Erdő. (2019). Overcoming the Blood–Brain Barrier. Challenges and Tricks for CNS Drug Delivery. Sci. Pharm.. 87, 6;
- Débora Braga Vieira, Lionel Fernel Gamarra. (2018). Multifunctional Nanoparticles for Successful Targeted Drug Delivery across the Blood-Brain Barrier. Molecular Insight of Drug Design;
- Jean-Christophe Olivier. (2005). Drug transport to brain with targeted nanoparticles. Neurotherapeutics. 2, 108-119;
- Franciska Erdő, Luca Anna Bors, Dániel Farkas, Ágnes Bajza, Sveinbjörn Gizurarson. (2018). Evaluation of intranasal delivery route of drug administration for brain targeting. Brain Research Bulletin. 143, 155-170;
- Dhrisya Chenthamara, Sadhasivam Subramaniam, Sankar Ganesh Ramakrishnan, Swaminathan Krishnaswamy, Musthafa Mohamed Essa, et. al.. (2019). Therapeutic efficacy of nanoparticles and routes of administration. Biomater Res. 23;
- Christine E. Krewson, Michele L. Klarman, W. Mark Saltzman. (1995). Distribution of nerve growth factor following direct delivery to brain interstitium. Brain Research. 680, 196-206;
- Zian Wang, Guojun Xiong, Wai Chun Tsang, Andreas G. Schätzlein, Ijeoma F. Uchegbu. (2019). Nose-to-Brain Delivery. J Pharmacol Exp Ther. 370, 593-601;
- L. V. Rubis. (2018). CHARACTERISTICS OF THE CAUSATIVE AGENTS OF ACUTE RESPIRATORY VIRAL INFECTIONS AND THE IMPORTANCE OF DISINFECTION MEASURES IN PREVENTING THEIR SPREAD. Det. infekc.. 17, 34-40.
https://telegra.ph/Coronaviruses--Coronaviridae-1968-09-03
@tribulechat_bot