Lora Spreading Factor
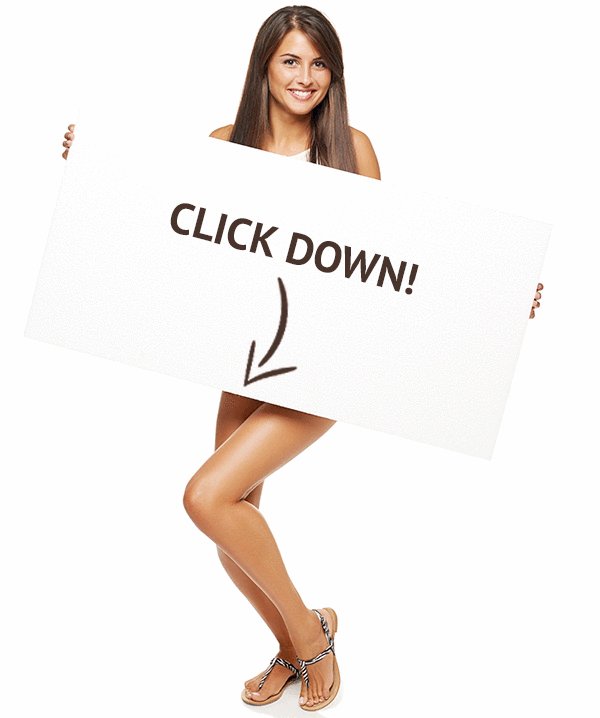
🔞 ALL INFORMATION CLICK HERE 👈🏻👈🏻👈🏻
Lora Spreading Factor
Выбрать язык русский азербайджанский аймара албанский амхарский арабский армянский ассамский африкаанс бамбара баскский белорусский бенгальский бирманский болгарский боснийский бходжпури валлийский венгерский вьетнамский гавайский галисийский греческий грузинский гуарани гуджарати датский догри зулу иврит игбо идиш илоканский индонезийский ирландский исландский испанский итальянский йоруба казахский каннада каталанский кечуа киргизский китайский (традиционный) китайский (упрощенный) конкани корейский корсиканский коса креольский (гаити) крио курдский (курманджи) курдский (сорани) кхмерский лаосский латинский латышский лингала литовский луганда люксембургский майтхили македонский малагасийский малайский малаялам мальдивский мальтийский маори маратхи мейтейлон (манипури) мизо монгольский немецкий непальский нидерландский норвежский ория оромо панджаби персидский польский португальский пушту руанда румынский самоанский санскрит себуанский сепеди сербский сесото сингальский синдхи словацкий словенский сомалийский суахили сунданский таджикский тайский тамильский татарский телугу тигринья тсонга турецкий туркменский узбекский уйгурский украинский урду филиппинский финский французский фризский хауса хинди хмонг хорватский чви чева чешский шведский шона шотландский (гэльский) эве эсперанто эстонский яванский японский
One Stop For Your RF and Wireless Need
This page describes LoRaWAN Spreading factor, range and data rate used in LoRa System.
The table and formulas for relation between Spreading factor vs distance range vs data rate are mentioned.
These terms are related to each other and they are explained below.
The terms are associated with chirp spread spectrum (CSS) modulation technique.
It uses wideband linear frequency modulated chirp pulses in order
to encode baseband information.
Chirp Spread Spectrum is used in space and military communications due to
its long range, low transmit power and less interference.
LoRa Modulation in LoRaWAN uses chirp spread spectrum for encoding.
Here, each bit is spread by chipping factor.
The number of chips per bit is known as spreading factor.
In other words, the duration of chirp is known as spreading factor.
CSS uses spreading factors from 7 to 12. The spreading factor for various regions
across the world are mentioned in the following tables.
As mentioned small spreading factors
offer high bit rate or LoRaWAN data rate and need less OTA (Over The Air) time.
Large spreading factors offer low data rates and need more over the air time.
In the table "RFU" refers to "Reserved for Future Usage".
The range is the distance covered by LoRaWAN signal to serve multiple end devices from single tower.
From following equation of free space path loss, LoRaWAN range can be easily derived.
As per one experiment, LoRaWAN range (i.e. distance) of 702.676 km was achieved using only
25 mW (i.e. 14dBm) of transmit power across border of Germany.
The LoRaWAN gateway is installed at the height of 30 meters.
As we know, data rate refers to rate at which data is transmitted from one one end to the
other through wired medium or wireless medium.
➨ Data rate = Number of bits transferred / Total time taken for transfer
Different wireless technologies will have data rate range different based on their
physical layer parameters (modulation, code rate etc.) and radio parameters
(bandwidth, number of carriers etc.) . LoRaWAN is also a wireless system.
The LoRaWAN data rate depending on Spreading factor, Bandwidth and code rate as per
equation mentioned below.
Following equation mentions relation between LoRa data rate,
LoRa spreading factor, Code Rate and Bandwidth.
Refer LoRa calculator >> which mentions calculation of LoRaWAN data rate
as per LoRaWAN spreading factor entered along with code rate and bandwidth.
©RF Wireless World 2012, RF & Wireless Vendors and Resources, Free HTML5 Templates
Spreading Factor, a key characteristic in LoRaWAN that can make or break your IoT solution. Finding the right Spreading Factor is crucial for realizing long-term performance of a LoRaWAN device. This article explains how to find the right balance between battery performance and long-range communication.
Spreading Factor (SF) decides on how many chirps, the carrier of the data, are sent per second. The network decides the spreading factor (graded between 7-12) based on the environmental conditions between the communication device and the gateway. This assumes that the Adaptive Data Rate functionality is switched on, which is recommended for all but the continuously moving devices.
Lower SF means more chirps are sent per second; hence, you can encode more data per second. Higher SF implies fewer chirps per second; hence, there are fewer data to encode per second. Compared to lower SF, sending the same amount of data with higher SF needs more transmission time, known as airtime. More airtime means that the modem is up and running longer and consuming more energy.
The benefit of high SF is that more extended airtime gives the receiver more opportunities to sample the signal power which results in better sensitivity. Better sensitivity means that you can receive the signal further away, so you get better coverage. Theoretically, each step up in SF doubles the time to transmit the same amount of data, see fig 1. Each step up in SF correlates to about 2.5dB extra link budget.
Fig.1 Relationship between spreading factor and airtime for LoRa modulation.
The theory is all great, but how does the airtime and battery life look like in practice?
To get a sense of this, we used two tools:
1. Airtime calculator from LoRaTools
2. Otii to measure energy consumption profiles for SF7 and SF12
In this test, we measured a LoRaWAN device from Bintel which uses Semtech's SX1276 chipset . Our set-up was an indoor-outdoor scenario, i.e. device was measured in an office, at a developer’s table, with a gateway outside on one of the buildings nearby. Hence, it was not the normal use scenario for this device, that is meant for waste bins, outdoors. We measured the energy consumption of the first transmission and total activity cycle with a payload of 19 bytes and a bandwidth of 125 kHz.
Fig. 2. Bintel LoRaWAN device for waste management.
In the measurement, the activity cycle comprised of a transmit and a listen period with a confirmed message, an acknowledgment (ack), see fig 3.
Fig.3. Measured energy consumption for transmission at SF12 (in blue) and SF7 (in green), with acknowledgement (ack) from the gateway.
Table I. Measured and calculated transmission time and measured energy consumed for SF12 and SF7.
The measurement shows that it takes approximately 25 times longer and 25 times more energy to transmit in SF12 compared to SF7. Airtime calculator shows the same result.
The number 25 is, however, not set in stone. It depends on the payload size and the headers assigned for the transmission. If you play with different sizes of the payload, you can see different multiples.
Note that the above calculation is for the transmit time only, and not for the whole activity cycle. The ratio of energy consumption per activity cycle is 20 times more energy for SF12 than SF7, in this specific measurement (energy stats showing 55.1 uWh vs. 2.78 uWh, see Fig.4). It is good to note that the energy consumption for the activity cycle depends on what Class the device is. If it is a Class A device, it has only two receive opportunities, as seen in the Fig. 5 (red trace), meaning the receiver is not awake more than for those two opportunities. Another configuration can be, for example, that the device keeps listening continuously, which increases the energy consumption drastically. In that case, the SF number doesn't matter.
Fig 4. Marked energy consumption for transmission at SF12 (in blue) and SF7 (in green), for the full activity cycle including uplink and downlink.
Fig 5. Measured energy consumption for transmission at SF12 (in red) and SF7 (in yellow), with no acknowledgement from the gateway.
It's difficult to draw more conclusions as each application is unique, but we want to highlight that the optimal spreading factor that will yield the lowest energy consumption is not necessarily the highest or the lowest one, but most likely somewhere in between. If there are excessive retries, due to the need for confirmed messages, the energy savings of a shorter transmission will rapidly get lost due to retransmissions. The important thing to remember is that the total energy consumption is never optimized with one parameter only.
To check out the energy measurements for different SFs presented, download .otii file below (and open it in Otii application that you can download here for free).
Do you want to learn more about optimizing the power consumption of your LoRaWAN deployment and meet the Qoitech experts in person? Join 2000 LoRaWAN professionals at The Things Conference on January 30 and 31, 2020 in Amsterdam; the world’s largest LoRaWAN conference.
GET YOUR TICKETS NOW
10% discount code: FRIEND-OF-QOITECH
You can download .otii file of the above measurements HERE
For more information about LoRa, LoRaWAN or The Things Network, have a look at one of the links below
- LoRa CHIRP
- LoRa crash course
- Decoding the LoRa PHY
Already have a WordPress.com account? Log in now.
Josef Matondang
Customize
Follow
Following
Sign up
Log in
Copy shortlink
Report this content
View post in Reader
Manage subscriptions
Collapse this bar
Email (Required)
Name (Required)
Website
In the previous article, I discussed about several basic Spread Spectrum concepts while specifically talking about LoRa modulation and touching the topic regarding several parameters in LoRa. Those parameters in question are Spreading Factor, Bandwidth, and Coding Rate. The three parameters will determine how sensitive the LoRa receiver will perform and how fast the data transmission speed will be. I will shortly discuss them in this article, hoping that the readers will be able to understand the concept and implement it in a LoRa-based system
As discussed before, LoRa is a chirp spread spectrum modulation. The transmitted data, which is a symbol, will be represented by a chirp signal with a frequency range from to , which is shown in Figure 1. In LoRa modulation, we can configure the symbol by changing the Spreading Factor and Bandwidth parameters. According to Application Note Semtech AN1200.22 , one symbol will take of second to transmit, which is a function of Bandwidth and Spreading Factor can be shown with the equation below:
Bandwidth is the frequency range of the chirp signal used to carry the baseband data. In Figure 1, the Bandwidth can be seen from the width of frequency used between to . Aside from that, Bandwidth can also represent chip rate from LoRa signal modulation
The value of Spreading Factor (SF) determines how many chips used to represent a symbol. The higher the SF value is, the more chips used to represent a symbol, which means there will be more processing gain from the receiver side. This will allow receiver to accept data signals with negative SNR value
Spreading Factor shows how many chips used to represent a symbol, with an exponential factor of 2. 1 symbol may consist of N chip where . A cyclic shift can be done to represent a bit and sent symbol. If there is N amount of chips, then the resulting symbol value may range from 0 to N-1, or that 1 symbol may represent SF bits
LoRa modulation also adds a forward error correction (FEC) in every data transmission. This implementation is done by encoding 4-bit data with redundancies into 5-bit, 6-bit, 7-bit, or even 8-bit. Using this redundancy will allow the LoRa signal to endure short interferences. The Coding Rate (CR) value need to be adjusted according to conditions of the channel used for data transmission. If there are too many interference in the channel, then it’s recommended to increase the value of CR. However, the rise in CR value will also increase the duration for the transmission
Fill in your details below or click an icon to log in:
You are commenting using your WordPress.com account.
( Log Out /
Change )
You are commenting using your Twitter account.
( Log Out /
Change )
You are commenting using your Facebook account.
( Log Out /
Change )
Notify me of new comments via email.
URL: https://www.sciencedirect.com/science/article/pii/B9780128188804000107
URL: https://www.sciencedirect.com/science/article/pii/B9780128188804000041
URL: https://www.sciencedirect.com/science/article/pii/B9780128193020000031
URL: https://www.sciencedirect.com/science/article/pii/B9780080999692000089
(6.1) C ⌢ i = C i T u , i = 1 , … , N L .
(6.2) S i = C i + ( S ˆ i − C ˆ i ) u , i = 1 , … , N L .
URL: https://www.sciencedirect.com/science/article/pii/B9780120471447500066
URL: https://www.sciencedirect.com/science/article/pii/B978012818880400003X
URL: https://www.sciencedirect.com/science/article/pii/B9780080999692000107
URL: https://www.sciencedirect.com/science/article/pii/B9780080999692000065
URL: https://www.sciencedirect.com/science/article/pii/B9780123983268000029
(5.83) x l ( i + 1 ) = x l ( i ) − μ I M ( i ) z l ( i ) P l r ( i ) r T ( i ) P l r ( i )
URL: https://www.sciencedirect.com/science/article/pii/B9780123741967000118
Spreading factors vary from 256 to 4 for uplink and from 512 to 4 for downlink, which allow data rates of up to 2 Mbps.
Daniele Croce , ... Ilenia Tinnirello , in LPWAN Technologies for IoT and M2M Applications , 2020
The SF allocation and distribution among the terminals can influence the capacity of a LoRa cell. Indeed, capture effects and inter-SF collisions depend on the power ration of the signals, which is obviously influenced by the relative position of the terminals. For example, let us consider inter-SF collisions between two SFs, without channel captures for the sake of simplicity. Generally, higher SFs are allocated to nodes further away from the gateway. Therefore, suppose that spreading factor SF 1 < SF 2 is assigned to users close to the gateway, up to a certain distance d (with r < d < R ), while SF 2 is assigned to users with distance r greater than d , that is, d < r < R .
In this scenario, and ignoring possible fading effects, users with spreading factor SF 1 will never be interfered by nodes with SF 2 because these users are located deterministically at greater distances, and thus the power received at the gateway will always be lower. Therefore, the performance obtained by SF 1 will be S 1 = G 1 ⋅ e − 2 ⋅ G 1 , as if the SFs were perfectly orthogonal. Vice versa, terminals using spreading factor SF 2 will likely be affected by nodes using SF 1 as these terminals are closer to the gateway, and thus the interference density will be higher than in the previous uniform allocation case. The previous throughput equations can be easily extended with the nonuniform load density functions δ 2 = G 2 /( πR 2 − πd 2 ) and δ 1 = G 1 /( πd 2 ).
Congduc Pham , ... Muhammad Ehsan , in LPWAN Technologies for IoT and M2M Applications , 2020
The spreading factor SF in LoRa is the ratio between symbol rate R s and chip rate R c : S F = l o g 2 ( R c / R s ) . LoRa employs six spreading factors from 7 to 12 that provide some sort of orthogonality in data transmission (collision-free) occurring on the same frequency. This orthogonality property can be explained as follows: different SF will give a different chirp rate, which is the change in the frequency with respect to time. As CSS uses frequency chirps with linear variation of frequency over time, when plotting frequency against time the chirp rate will be the slope of the line. Therefore, theoretically, different SF will give different slopes that provide the orthogonality property.
However, when using a LoRa combination of B W and SF , not all combinations are orthogonal because some of them define the same chirp rate (same slope) [7] . This can be further explained as follows: the slope (chirp rate) can be defined as slope = ( f max − f min ) / T s , where T s is the symbol duration. As the symbol rate is R s = B W / 2 S F then T s = 1 / R s = 2 S F / B W . With f max − f min = B W we have slope = B W * ( B W / 2 S F ) = B W 2 / 2 S F . For instance, if we take SF7BW125, SF9BW250, and SF11BW500, we have the same chirp rate that is 122070312.5. Fig. 4–10 shows all BW and SF combinations indicating those that are not orthogonal by an “x.”
Figure 4–10 . Orthogonality of SF and B W combination. x=not orthogonal.
It is also worth mentioning that [8] reported some issues with different SF values when there is high power difference such as in near-far conditions.
Yusheng Shi , ... Ying Chen , in Materials for Additive Manufacturing , 2021
The contact angle, maximum spreading factor and spreading time of the support material on its cured surface and the solid surface of the cationic solid material are shown in Table 3.29 (50°C); the contact angle, spreading factor and spreading time of the support material with the hybrid solid material and the free-radical solid material are shown in Tables 3.30 and 3.31 (50°C), respectively. It can be seen from the table that the spreading of the support material on the cured surface of various solid materials is generally the same, there is no big difference, and the spreading factor is not very large compared with the spreading factor of the solid material.
Table 3.29 . Contact angle, spreading factor and spreading time of support materials on cationic solid materials.
Table 3.30 . Contact angle, spreading factor and spreading time of support materials on hybrid solid materials.
Table 3.31 . Contact angle, spreading factor and spreading time of support materials on free-radical solid materials.
Thomas Chapman , ... Johan Sköld , in HSPA Evolution , 2015
The HS-SCCH, sometimes referred to as the shared control channel , is a shared downlink physical channel that carries control signaling information needed for a UE to be able to properly despread, demodulate, and decode the HS-DSCH.
In each 2 ms interval corresponding to one HS-DSCH TTI, one HS-SCCH carries physical-layer signaling to a single UE. As HSDPA supports HS-DSCH transmission to multiple users in parallel by means of code multiplexing (see Section 8.1.1 ), multiple HS-SCCH may be needed in a cell. According to the specification, a UE should be able to decode four HS-SCCHs in parallel. However, more than four HS-SCCHs can be configured within a cell, although the need for this is rare.
HS-SCCH uses a spreading factor of 128 and has a time structure based on a subframe of length 2 ms that is the same length as the HS-DSCH TTI. The following information is carried on the HS-SCCH:
The HS-DSCH transport format, consisting of:
HS-DSCH channelization-code set [7 bits];
HS-DSCH m
Rimming Sperm
Art Lingerie Hd
Anus Spreading