In Autosomal Dominant Inheritance Php Gal
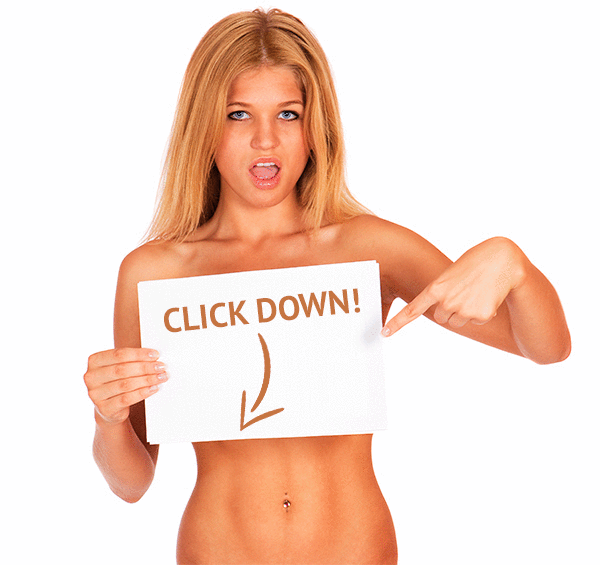
🛑 ALL INFORMATION CLICK HERE 👈🏻👈🏻👈🏻
In Autosomal Dominant Inheritance Php Gal
This person is not on ResearchGate, or hasn't claimed this research yet.
Inherited retinal diseases (IRDs) have been in the front line of gene therapy development for the last decade, providing a useful platform to test novel therapeutic approaches. More than 40 clinical trials have been completed or are ongoing, tackling autosomal recessive and X-linked conditions, mostly through adeno-associated viral vector delivery of a normal copy of the disease-causing gene. However, only recently has autosomal dominant (ad) disease been targeted, with the commencement of a trial for rhodopsin ( RHO )-associated retinitis pigmentosa (RP), implementing antisense oligonucleotide (AON) therapy, with promising preliminary results ( NCT04123626 ).
Autosomal dominant RP represents 15%–25% of all RP, with RHO accounting for 20%–30% of these cases. Autosomal dominant macular and cone-rod dystrophies (MD/CORD) correspond to approximately 7.5% of all IRDs, and approximately 35% of all MD/CORD cases, with the main causative gene being BEST1 . Autosomal dominant IRDs are not only less frequent than recessive, but also tend to be less severe and have later onset; for example, an individual with RHO -adRP would typically become severely visually impaired at an age 2–3 times older than in X-linked RPGR -RP.
Gain-of-function and dominant negative aetiologies are frequently seen in the prevalent adRP genes RHO , RP1 and PRPF31 among others, which would not be effectively addressed by gene supplementation alone and need creative, novel approaches. Zinc fingers, RNA interference, AON, translational read-through therapy, and gene editing by clustered regularly interspaced short palindromic repeats/Cas are some of the strategies that are currently under investigation and will be discussed here.
Disease mechanisms and therapeutic approaches for autosomal dominant inherited retinal dystrophies (IRDs). (A) Normal. We see the normal process of DNA transcription to messenger RNA (mRNA), and then RNA translation to protein. The wild-type gene is depicted in yellow and its promoter in a darker shade. Normal proteins are seen in pink, two of them bound to their receptor. (B) Loss-of-function (LOF), where the gene is seen in red. In this case, the mRNA transcript is shorter due to a null disease-causing variant prematurely stopping transcription, consequently halting translation and leading to truncated/ absent protein. The yellow squares represent the therapeutic approaches under development to treat LOF IRDs, at the location where they have their therapeutic effect. *Corresponds to the only mechanism currently approved to treat LOF RPE65-associated retinal dystrophy. (C) Gain-offunction (GOF), with the gene in blue. We see abnormal protein formed (light blue), toxic to the cell, and the yellow squares representing therapeutic avenues. (D) Dominant negative effect (DNE), with a light green gene. In this situation, abnormal proteins (green) compete with the wild-type for binding receptors. In yellow, therapeutic mechanisms. AON, antisense oligonucleotides; CRISPR, clustered regularly interspaced short palindromic repeats. on August 30, 2022 at UCL Library Services. Protected by copyright.
Gene therapy strategies currently being investigated for autosomal dominant inherited retinal dystrophies
All figure content in this area was uploaded by Malena Daich Varela
Content may be subject to copyright.
Content uploaded by Malena Daich Varela
Content may be subject to copyright.
Daich VarelaM, etal . Br J Ophthalmol 2022; 0 :1–8. doi:10.1136/bjophthalmol-2022-321903
Genetic treatment for autosomal dominant inherited
retinal dystrophies: approaches , challenges and
Malena Daich Varela , 1,2 T assos Georgiadis, 3 Michel Michaelides 1,2
Inherited retinal diseases (IRDs) have been in the front
line of gene therapy development for the last decade,
providing a useful platform to test novel therapeutic
approaches. More than 40 clinical trials have been
completed or are ongoing, tackling autosomal recessive
and X- linked conditions, mostly through adeno-
associated viral vector delivery of a normal copy of
the disease- causing gene. However , only recently has
autosomal dominant (ad) disease been targeted, with
the commencement of a trial for rhodopsin ( RHO )-
associated retinitis pigmentosa (RP), implementing
antisense oligonucleotide (AON) therapy, with promising
Autosomal dominant RP represents 15%–25% of all
RP , with RHO accounting for 20%–30% of these cases.
Autosomal dominant macular and cone- rod dystrophies
(MD/CORD) correspond to approximately 7.5% of all
IRDs, and appro ximately 35% of all MD/CORD cases,
with the main causative gene being BEST1 . Autosomal
dominant IRDs are not only less frequent than recessive,
but also tend to be less severe and have later onset; for
example, an individual with RHO - adRP would typically
become severely visually impaired at an age 2–3 times
older than in X- linked RPGR - RP .
Gain- of- function and dominant negative aetiologies
are frequently seen in the prevalent adRP genes RHO ,
RP1 and PRPF31 among others, which would not be
effectively addressed by gene supplementation alone
and need creative, novel approaches . Zinc fingers, RNA
interference, AON, tr anslational read- through therapy,
and gene editing by clustered regularly interspaced short
palindromic repeats/Cas are some of the strategies that
are currently under investigation and will be discussed
The complex group of inherited retinal dystrophies
(IRDs) has been under the spotlight for the last two
decades. 1 2 The accessible ocular anatomy , relative
immune privilege, lack of photoreceptor mitosis,
state- of - the- art instruments to evaluate the retina,
nearly- exclusive monogenic aetiology and small
volume of the eye, have made IRDs a promising
field for the development of cutting- edge gene
Autosomal recessive and X - linked IRDs have
been the main therapeutic target, with gene supple -
mentation being the leading technique. 3 Over 40
clinical trials have been completed or are ongoing,
using mostly adeno- associated viral (AA V) vectors
to supply a normal copy of the disease- causing gene
and create a normal, fully functioning protein. In
2019, the first gene- specific nucleic acid thera -
peutic phase 1/2 trial for an autosomal dominant
(ad/AD) IRD started, recruiting individuals with
RHO P23H- related retinitis pigmentosa (RP;
NCT04123626). Preliminary results of improve -
ments in best- corrected visual acuity (BCV A) and
retinal sensitivity are promising (https://www .
proqr.com/files/2021-11/Analyst-Event-2021_
FOR-DOWNLOAD_OK.pdf). It is anticipated
that this will be the first of a new wave of clinical
trials for the large unmet need of treatments for
Here, we discuss the current clinical and preclin-
ical landscape of the therapeutic approaches for
ad- IRD, and prioritise the most investigated geno-
types and most likely to be translated to clinical
DOMINANT IRD AND POTENTIAL THERAPEUTIC
Autosomal dominant RP accounts for approximately
15%–22% of all RP . 4–6 The most common caus-
ative gene is rhodopsin ( RHO ), found in 20%–30%
of cases. 7–9 The missense p.(P23H) is the most
common variant, as well as the first point mutation
identified to cause adRP in humans. 7 10 Rhodopsin
is followed in frequency by PRPF31 (8%–10%), 11
RP1 (8%–10%), 8 12 PRPH2 (10%), 13 IMPDH1
(5–10%), 14 NR2E3 (1%–3.5%, with p.(G56R)
being the second most commonly associated variant
with adRP), 15 SNRNP200 (1.5%–2.3%), 9 16 and
AD MD/CORD account for approximately 7.5%
of IRD, and 34% of MD/CORD cases in total. 17 18
The main causative gene is BEST1 (3.5%), followed
by PRPH2 (2%) and then EFEMP1 , TIMP3 ,
GUCA1A , GUCY2D , PRDM13 , ELOVL4 and
PROM1 , each with less than 1% frequency . 17
Dominant conditions are not only less frequent
than recessive, but also tend to be less severe. Indi-
viduals with RHO - related RP are reported to reach
legal blindness at a mean age of 79 years old. 19 While
in patients with recessive USH2A - RP , this occurs at
a median of 58 years old, 20 and in X- linked RPGR -
RP , by the third to fourth decade of life. 21 Patients
with Best disease ( BEST1 ) can maintain good BCV A
over time, often showing no significant differences
between baseline and follow- up acuity in longitu-
dinal studies. 22 23 On the other hand, individuals
with recessive ABCA4 - related Stargardt disease,
on August 30, 2022 at UCL Library Services. Protected by copyright. http://bjo.bmj.com/ Br J Ophthalmol: first published as 10.1136/bjo-2022-321903 on 29 August 2022. Downloaded from
2 Daich VarelaM, etal . Br J Ophthalmol 2022; 0 :1–8. doi:10.1136/bjophthalmol-2022-321903
often lose three or more Early T reatment Diabetic Retinopathy
Study (ETDRS) lines over 10 years' time. 24
A challenge that AD conditions face is that haploinsufficiency
is rarely their mechanism of disease. Gain- of- function and domi-
nant negative aetiologies are frequently seen in the most preva-
lent AD genes: RHO , RP1 and PRPF31 , among others. 25 These
cannot be treated by gene supplementation alone and need
creative, novel approaches that are in the early stages of first in
man testing ( table 1 ). These methodologies ( figure 1 ) include:
► Zinc fingers (ZFs), proteins that bind promoters and func-
tion as artificial transcription factors, enhancing/supressing
► Antisense oligonucleotides (AONs), single- stranded RNA or
DNA molecules that bind pre- mRNA or mRNA, and alter its
splicing and/or block translation. 27
► RNA interference (RNAi), a naturally occurring pathway
that identifies viral RNA and prevents their translation
through: (1) short- interfering RNA (siRNA), highly selective
double- stranded complex that binds and cleaves mRNA;
(2) microRNA (miRNA), single- stranded RNA molecules
that commonly bind to the 3’ untranslated region and block
mRNA translation 27 ; and (3) short- hairpin RNA (shRNA),
double- stranded RNA sequences linked by a short loop,
capable of DNA integration, which are subsequently trans-
formed into siRNA in the cytosol. 28
► T ranslational read- through therapy , an approach applicable
for nonsense point mutations where drugs bind to ribosomes
and force translation beyond the erroneous stop codon,
leading to a full- length protein. 29 30
► And CRISPR (clustered regularly interspaced short palin-
dromic repeats)/Cas genome editing system, correcting
disease- causing variants in native alleles. 31
RHO encodes rhodopsin, a G protein- coupled receptor located
in the disc membrane of rod outer segments, which is the first
component of the phototransduction cascade. 32 RHO - related
retinopathy is common, with a well understood molecular
basis. It can be classified either according to the genotype
or phenotype. Sung et al divided the causative variants into
two classes according to their biochemical properties: Class
I - accumulating in the plasma membrane and resembling the
wild- type regarding regeneration of 11- cis- retinal; and class
II—with variable regeneration of the chromophore and accu -
mulation in the endoplasmic reticulum. 33 Cideciyan et al clas -
sified it on the basis of disease severity , where class A presents
with severe, widespread loss of rods, and class B corresponds
to sector RP , often involving the inferior retina. 34 Genotype–
phenotype correlations have been attempted, with a relation -
ship observed between rhodopsin destabilisation and phenotype
severity . However, disease often presents with markedly vari -
able severity , even within families, indicating possible epigenetic
Table 1 Gene therapy strategies currently being investigated for autosomal dominant inherited retinal dystrophies
Gene Variant Mechanism (drug) Route of delivery Status
RHO P23H Antisense oligonucleotide (QR- 1123) Intravitreal Phase I/II CT (NCT04123626; ProQR T herapeutics)
RHO P23H Autophagy reduction (hydroxychloroquine) Oral Phase I/II CT (NCT04120883; University of Michigan)
RHO P23H Transgenic gene supplementation – Mouse models 45
RHO Unspecific Zinc fingers Subretinal Mouse models 26
RHO Unspecific Ectopic silencing transcription factor (KLF15) Subretinal Mouse models 46
RHO P347S Coadministration of two AAV containing RNAi and a codon-
RHO P23H Administration of one AAV containing both siRNA and a codon-
RHO P347S Administration of shRNA- expressing AAV and an AA V
expressing shRNA- resistant rhodopsin
RHO P23H and D190N Ablate- and- replace strategy, with dual AAV injection of CRISPR/
RHO S334 Allele- specific ablation using CRISPR/Cas9 with targeting- guide
RHO P23H AAV delivered CRISPR/Cas9 with short guide RNA Intravitreal Mouse models and human cells 53
RHO P23H AAV delivered CRISPR/Cas9 with short guide RNA Subretinal Mouse models 54
RHO P23H Antisense oligonucleotide Intravitreal Mouse and rat models 55
RHO S334 Aminoglycoside read- through (gentamicin or geneticin) Subcutaneous Rat models 56
PRPF31 Unspecific AAV - mediated gene augmentation – Induced pluripotent stem cells - RPE cells 71
PRPH2 Unspecific Nanoparticles containing wild- type PRPH2 Subretinal Mouse models 93
PRPH2 Unspecific AAV - delivered siRNAs and resistant PRPH2 Subretinal Mouse models 95
PRPH2 Unspecific si/shRNAs and resistant PRPH2 – Retinal organotypic culture 96
PRPH2 Unspecific AAV- delivered shRNAs Subretinal Mouse models 97
IMPDH1 Unspecific AAV - delivered shRNA and resistant IMPDH1 Subretinal Mouse models 102
BEST1 R218H, 234P , A243T , 293K and D302A AAV- mediated gene augmentation – iPSC- RPEs 110
BEST1 D203A, I205T and Y236C Baculovirus- based silencing vector delivery of CRISPR/Cas9 and
BEST1 R218C and N296H Lentivirus mediated gene augmentation – iPSC- RPE 117
BEST1 R218C, N296H and A146K Lentivirus construct delivery of CRISPR/Cas9 – iPSC- RPE 117
AAV , adeno- associated virus; CRISPR, clustered regularly interspaced short palindromic repeats; CT , clinical trial; iPSC, induced pluripotent stem cells; RNAi, RNA interference; RPE, retinal pigment
epithelium; shRNA, short- hairpin RNA; siRNA, short- interfering RNA.
on August 30, 2022 at UCL Library Services. Protected by copyright. http://bjo.bmj.com/ Br J Ophthalmol: first published as 10.1136/bjo-2022-321903 on 29 August 2022. Downloaded from
Daich VarelaM, etal . Br J Ophthalmol 2022; 0 :1–8. doi:10.1136/bjophthalmol-2022-321903
RHO - adRP is characterised by a slow rate of progression
(particularly Class B), posing a challenge when determining
clinical endpoints. It has been suggested that a vertical foveal
photoreceptor and retinal pigment epithelium (RPE) band thick-
ness and ellipsoid zone (EZ) width may be possible outcome
measures, 37 as well as the hyperautofluorescent ring diameter
seen on short wavelength- autofluorescence. 37–39
The most common disease- causing variants in RHO are gain-
of- function and have a dominant negative effect. 40 41 This means
that the defective protein is retained intracellularly , inducing
the unfolded protein response and the degradation of both the
abnormal and wild- type protein. Animal models resembling the
human disease have been successfully achieved in mice, 42 43 setting
the basis for testing novel preclinical therapeutic approaches. 44 A
natural history study for RHO - RP is currently active and taking
place in USA and France (NCT04285398, table 2 ).
Many techniques have been explored to treat RHO -
retinopathy . Price et al have used the somewhat classical tech-
nique of AA V- associated gene supplementation in P23H mice,
and found that the retinal degeneration persisted, suggesting
that excessive amounts of rhodopsin alone cannot rescue photo-
receptors. 25 45 AA V- delivered ZFs have also been employed,
targeting the RHO promoter, and were associated with
mutation- unspecific decreased translation and improved disease
in a mouse model. 26 Another method to interfere with promoter
function that has been tested is through AA V- mediated ectopic
expression of a transcription factor capable of silencing RHO
(KLF15), with structural and functional protection observed in
Post- transcriptional protein knockdown has also been
attempted through hammerhead and hairpin ribozymes designed
to target and cleave P23H, with good specificity in vitro. 47 In
addition, a dual- approach to both suppress the mutated gene
and supplement a wild- type gene is being actively developed.
Suppression has been implemented via RNA silencing (eg, RNAi
and siRNA) 48–50 and CRISPR/Cas9, 51 combined with gene
supplementation (RNAi- resistant where applicable), leading to
visual function improvement in mouse models. This was assessed
by electrophysiology , where rod- isolated responses improved
significantly post- treatment, and by histology , with preserva-
tion of the outer nuclear layer (ONL) and the outer segments
of photoreceptors. 48–51 Different groups have tried allele
specific CRISPR/Cas9 editing alone, with visual acuity (V A) and
retinal function improvement in Rho S334 and Rho +/P23H mouse
models. 52–54 RNA knockdown alone has also shown significant
improvement in retinal function and structure in P23H rats and
mice. 55 The latter has led to a phase I/II clinical trial of AON
and targets patients with P23H RHO - RP (NCT04123626), with
T ranslational read- through drugs have also been tested in
RHO S334ter rat models, with an increased number of surviving
photoreceptors and improved electroretinography (ERG) record-
ings. 56 Gregory- Evans et al tested the use of a read- through drug
combined with neuroprotection in the same rats, and found
indistinguishable histology from unaffected controls. 57
Neuroprotection has also been investigated with a subret -
inal injection of an AA V vector expressing a glial cell line
derived neurotrophic factor, which was resulted in preserva -
tion of ONL thickness and increased ERG responses in mouse
models. 58 Lastly , Yao et al found that reducing autophagy in
P23H photoreceptors through hydroxychloroquine oral treat -
ment and/or deletion of the autophagic gene ATG5 , decreased
cell death in mice and had a protective effect. 59 This led to
another ongoing clinical trial (NCT04120883), which uses oral
Figure 1 Disease mechanisms and therapeutic approaches for
autosomal dominant inherited retinal dystrophies (IRDs). (A)Normal . We
see the normal process of DNA transcription to messenger RNA (mRNA),
and then RNA translation to protein. The wild- type gene is depicted
in yellow and its promoter in a darker shade. Normal proteins are
seen in pink, two of them bound to their receptor . (B)Loss- of- function
(LOF), where the gene is seen in red. In this case , the mRNA transcript
is shorter due to a null disease- causing variant prematurely stopping
transcription, consequently halting translation and leading to truncated/
absent protein. The yellow squares represent the therapeutic approaches
under development to treat LOF IRDs, at the location where they have
their therapeutic effect. *Corresponds to the only mechanism currently
approved to treat LOF RPE65 - associated retinal dystrophy. (C)Gain- of-
function (GOF), with the gene in blue. We see abnormal protein formed
(light blue), toxic to th
Girls Taking Nude Selfies
Mario Is Missing Hentai Game
Try Not To Get A Hard On