Sigal Mfc
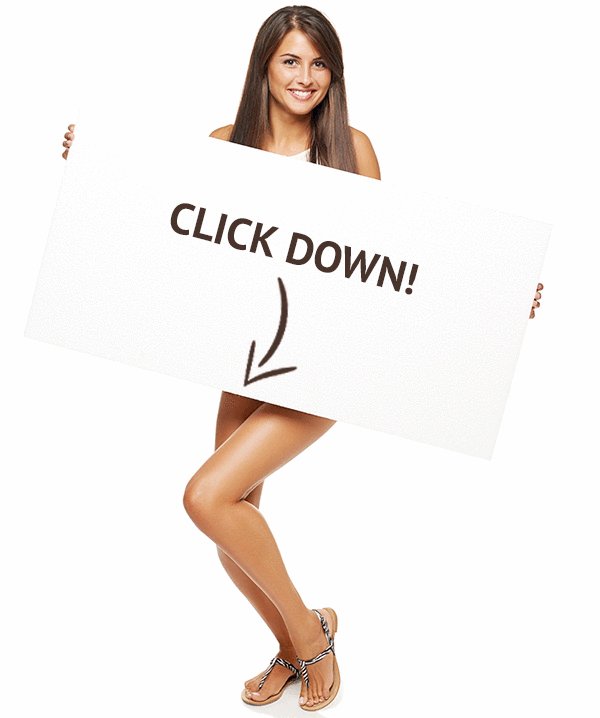
⚡ ALL INFORMATION CLICK HERE 👈🏻👈🏻👈🏻
Sigal Mfc
We select and review products independently. When you purchase through our links we may earn a commission. Learn more.
Jan 12, 2021, 6:40 am EDT
| 4 min read
How-To Geek is where you turn when you want experts to explain technology. Since we launched in 2006, our articles have been read more than 1 billion times. Want to know more?
Join 425,000 subscribers and get a daily digest of news, geek trivia, and our feature articles.
By submitting your email, you agree to the Terms of Use and Privacy Policy .
Chris Hoffman is Editor-in-Chief of How-To Geek. He's written about technology for over a decade and was a PCWorld columnist for two years. Chris has written for The New York Times and Reader's Digest , been interviewed as a technology expert on TV stations like Miami's NBC 6, and had his work covered by news outlets like the BBC. Since 2011, Chris has written over 2,000 articles that have been read more than one billion times---and that's just here at How-To Geek. Read more...
Signal is a secure encrypted messaging app. Think of it as a more private alternative to WhatsApp, Facebook Messenger, Skype, iMessage, and SMS. Here’s why you should seriously consider switching to Signal.
Signal is available for Android, iPhone, and iPad. There’s also a Signal desktop client for Windows, Mac, and Linux. To join, all you need is a phone number. It’s free.
The user experience of Signal is just like WhatsApp, Facebook Messenger, and other popular chat apps. It’s a messaging app with features like one-to-one messages, groups, stickers, photos, file transfers, voice calls, and even video calls. You can have group chats with up to 1000 people and group calls with up to eight people .
Signal isn’t owned by a big tech company. Instead, Signal is developed by a non-profit foundation and is funded by donations . Unlike Facebook, Signal’s owners aren’t even trying to make money. Signal doesn’t try to gather a bunch of data on you or show you advertisements.
While Signal has a very familiar interface, it’s very different under the hood. Your conversations in Signal are end-to-end encrypted, which means that not even the owners of Signal can monitor them. Only the people in the conversation can see them.
Signal is also completely open-source . The source code for the project’s client apps and server software is available on GitHub .
All communications on Signal—including one-to-one messages, group messages, file transfers, photos, voice calls, and video calls—are end-to-end encrypted. Only the people involved in the communication can see them. The encryption happens between the individual devices using Signal. The company that operates Signal could not see these messages even if it wanted to. Signal actually created its own encryption protocol for this.
This is very different from traditional messaging apps. For example, Facebook has access to everything you say in Facebook Messenger. Facebook says it won’t use the content of your messages for advertising, but are you confident that will never change in the future?
Sure, some other messengers offer encrypted messaging as an optional feature. But everything on Signal is encrypted, always and by default. Signal also offers other privacy features, including self-destructing (disappearing) messages that will automatically be removed after a period of time.
Facebook Messenger collects a lot of data about you, too. Most companies collect a lot of data. Signal tries not to.
Even if Signal is subject to a subpoena on you and forced to disclose what it knows about you, the company knows almost nothing about you and your Signal activity. Signal could reveal only your account’s phone number, last connection date, and account creation time.
In contrast, Facebook could reveal your full name, everything you’ve said on Facebook Messenger, a list of geographical locations you’ve accessed your account from—and so on.
Everything in your Signal app—messages, pictures, files, and so on—is stored locally on your phone. You can manually transfer data between devices , but that’s it.
Signal’s end-to-end encryption is its big feature. That’s why so many people are using Signal—because they’re concerned about privacy. At the start of 2021, it’s been endorsed by everyone from Elon Musk to Twitter CEO Jack Dorsey and has shot to the top of Apple and Google’s app store charts.
But Signal didn’t come from nowhere—it was founded in 2013. It’s a widely respected piece of software that’s long been used by privacy advocates and other activists. Edward Snowden endorsed Signal back in 2015.
At the start of 2021, Signal has reached even greater mainstream acceptance. WhatsApp is revamping its privacy policy to share even more data with Facebook , and many people clearly want to bring their conversations out of Mark Zuckerberg’s view and embrace privacy.
While your communications on Signal are private, you aren’t anonymous. To sign up for Signal, you need a phone number. To talk to someone on Signal, your phone number is your identifier on Signal.
That’s by design—Signal is designed to be a drop-in replacement for SMS. When you sign up for Signal and install the app, it will ask for access to the contacts on your phone. Signal securely scans your contacts to see which of them are also Signal users—it just examines the phone numbers and sees if those phone numbers are also registered on Signal.
So, if you and someone else communicate via SMS, you can both install Signal and easily switch. If you install Signal, you can see which of your contacts you can message via Signal instead of SMS. You don’t have to ask them what their Signal handle is—it’s just their phone number. (However, you can verify the safety numbers associated with a conversation to ensure you’re talking directly with the person you think you are. That’s another useful security feature in Signal.)
If you’re concerned about other people you talk to on Signal having your phone number, you can try signing up with a secondary phone number . But, realistically, if you’re looking for a chat solution that isn’t dependent on phone numbers—for example, an anonymous chat solution that just uses usernames instead of phone numbers—then Signal isn’t what you’re looking for.
Signal is simple to get started with. Just download the official Signal app from either Apple’s App Store for iPhone and iPad or Google Play for Android . Go through the setup process to give Signal a phone number and access to your contacts. ( Contact access is optional , but Signal is designed to work best with it.)
You can then start conversations from within the app. If you have someone in your contacts and that person’s phone number is associated with a Signal account, you’ll see that you can contact them on Signal. It’s seamless.
Want to start talking with someone on Signal instead of a different chat app? Just ask them to download it and sign up. You’ll even get a notification when someone you know signs up for Signal .
You can also download the Signal desktop app for Windows, Mac, or Linux from the SIgnal Foundation’s website. This will sync messages from the Signal app on your phone to your computer. However, it’s optional.
The Best Free Tech Newsletter Anywhere
By submitting your email, you agree to the Terms of Use and Privacy Policy .
Dashboard
Publications
Account settings
Log out
Journal List
HHS Author Manuscripts
PMC3567446
Nanomedicine (Lond). Author manuscript; available in PMC 2013 Jul 1.
1 The Robert H. Smith Faculty of Agriculture, Food & Environment, the Hebrew University of Jerusalem, Israel
2 Department of Biomedical Engineering, Tufts University, 4 Colby Street, Medford, MA 02155, USA
* Author for correspondence: Tel.: +972 8 948 9084 Fax: +972 8 946 2283 li.ca.ijuh.irga@voyesohs
The publisher's final edited version of this article is available at Nanomedicine (Lond)
See other articles in PMC that cite the published article.
Keywords: bio-inspired, cellulose, collagen, resilin, silk, SP1
Cellulose is the most abundant biopolymer on earth and has traditionally been used for clothing, construction, furniture and for paper making for thousands of years.
In recent years, efforts have been made to produce nanostructured bio-inspired composites to mimic the features in natural cellulose composites.
Nanocrystalline cellulose (NCC) is defined as fibers which have been grown under controlled conditions that lead to the formation of high-purity single crystals.
The modulus of NCC is estimated to be approximately 150 GPa and its tensile strength is estimated to be approximately 10 GPa, similar to very strong materials such as aramid fibers (Kevlar®) and carbon fibers.
Polymeric proteins display diverse mechanical properties from high strength to elasticity, which are often superior to their synthetic counterparts.
Invertebrate skeletons are sophisticated multifunctional structures providing structural and mechanical support, shape, protection and complex locomotion.
Many of the skeleton proteins are bound to the chitin scaffold via a chitin-binding domain.
Resilin is a rubber-like protein that displays stretching ability up to 100% and is accompanied by a very high fatigue lifetime and low modulus of elasticity.
The high elasticity of resilin combined with the strength of the chitin in the composite enables insects to rapidly restore body shape as well as most of the energy required for the next mechanical movement (either flying or jumping).
Spider dragline silk, used as the safety line and as part of the frame thread of spider webs, is the strongest natural fiber known, reaching tensile strengths of 740 MPa.
Recombinant spider silks can be generated in many variants for biomaterials and tissue engineering.
Collagen architecture plays an important role in optimal functioning of various extracellular matrix-rich tissues, such as skin, tendons, muscle and cornea.
A significant development in the use of biomaterial-based biopolymers is in the field of regenerative medicine.
Collagen is the major component of the extracellular matrix and therefore it is a natural choice for applications in tissue engineering and regenerative medicine.
Natural composites such as plant cell walls, invertebrate skeletons and mammalian extracellular matrix present versatile mechanical properties ranging from high strength to high elasticity.
1. Werkmeister J, Ramshaw J. Recombinant protein scaffolds for tissue engineering. Biomed. Mater. 2012; 7 (1):012002. [ PubMed ] [ Google Scholar ]
2. Azami M, Moosavifar MJ, Baheiraei N, Moztarzadeh F, Ai J. Preparation of a biomimetic nanocomposite scaffold for bone tissue engineering via mineralization of gelatin hydrogel and study of mineral transformation in simulated body fluid. J. Biomed. Mater. Res. Part A. 2012; 100A (5):1347–1355. [ PubMed ] [ Google Scholar ]
3. Shoseyov O, Shani Z, Levy I. Carbohydrate binding modules: biochemical properties and novel applications. Microbiol. Mol. Biol. Rev. 2006; 70 (2):283–295. [ PMC free article ] [ PubMed ] [ Google Scholar ] ■ Carbohydrate-binding modules are essential components for self assembly of protein–polysaccharide composites.
4. Teeri T, Brumer H, Daniel G, Gatenholm P. Biomimetic engineering of cellulose-based materials. Trends Biotechnol. 2007; 25 (7):299–306. [ PubMed ] [ Google Scholar ]
5. Hubbe M, Rojas O, Lucia L, Sain M. Cellulosic nanocomposites: a review. Bio. Resour. 2008; 3 (3):929–980. [ Google Scholar ]
6. Berglund LA, Peijs T. Cellulose biocomposites – from bulk moldings to nanostructured systems. MRS Bulletin. 2010; 35 (3):201–207. [ Google Scholar ]
7. Eichhorn SJ, Dufresne A, Aranguren M, et al. Review: current international research into cellulose nanofibres and nanocomposites. J. Mater Sci. 2010; 45 (1):1–33. [ Google Scholar ] ■ Provides an overview of recent progress made in the area of cellulose nanofiber-based nanocomposites.
8. Siqueira G, Bras J, Dufresne A. Cellulosic bionanocomposites: a review of preparation, properties and applications. Polymers. 2010; 2 (4):728–765. [ Google Scholar ]
9. Rånby BG. Aqueous colloidal solutions of cellulose micelles. Acta Chem. Scand. 1949; 3 :649–650. [ Google Scholar ] ■ Describes for the first time the formation of nanocrystalline cellulose.
10. Favier V, Canova GR, Cavaille JY, Chanzy H, Dufresne A, Gauthier C. Nanocomposite materials from latex and cellulose whiskers. Polym. Adv. Technol. 1995; 6 (5):351–355. [ Google Scholar ] ■ Describes the dramatic improvement of mechanical properties of latex by small amounts of nanocrystalline cellulose.
11. Sakurada I, Nukushina Y, Ito T. Experimental determination of the elastic modulus of crystalline regions in oriented polymers. J. Polym. Sci. 1962; 57 (163):651–660. [ Google Scholar ]
12. Capadona J, Shanmuganathan K, Tyler D, Rowan S, Weder C. Stimuli-responsive polymer nanocomposites inspired by the sea cucumber dermis. Science. 2010; 319 (5868):1370–1080. [ PubMed ] [ Google Scholar ]
13. Tang L, Weder C. Cellulose whisker/epoxy resin nanocomposites. ACS Appl. Mat. Interf. 2010; 2 (4):1073–1080. [ PubMed ] [ Google Scholar ]
14. Rusli R, Shanmuganathan K, Rowan S, Weder C, Eichhorn S. Stress-transfer in anisotropic and environmentally adaptive cellulose whisker nanocomposites. Biomacromolecules. 2010; 11 (3):762–768. [ PubMed ] [ Google Scholar ]
15. Samir MASA, Alloin F, Dufresne A. Review of recent research into cellulosic whiskers, their properties and their application in nanocomposite field. Biomacromolecules. 2005; 6 (2):612–626. [ PubMed ] [ Google Scholar ]
16. Karouzou M, Spyropoulos Y, Iconomidou V, Cornman R, Hamodrakas S, Willis J. Drosophila cuticular proteins with the R&R consensus: annotation and classification with a new tool for discriminating RR-1 and RR-2 sequences. Insect Biochem. Mol. Biol. 2007; 37 (8):754–760. [ PubMed ] [ Google Scholar ]
17. Levy I, Shoseyov O. Cross bridging proteins in nature and their utilization in bio-and nanotechnology. Curr. Protein Pept. Sci. 2004; 5 (1):33. [ PubMed ] [ Google Scholar ]
18. Levy I, Nussinovitch A, Shpigel E, Shoseyov O. Recombinant cellulose crosslinking protein: a novel paper-modification biomaterial. Cellulose. 2002; 9 (1):91–98. [ Google Scholar ]
19. Levy I, Paldi T, Shoseyov O. Engineering a bifunctional starch–cellulose cross-bridge protein. Biomaterials. 2004; 25 (10):1841–1849. [ PubMed ] [ Google Scholar ]
20. Velema J, Kaplan D. Biopolymer-based biomaterials as scaffolds for tissue engineering. Adv. Biochem. Eng. Biotechnol. 2006; 102 :187–238. [ PubMed ] [ Google Scholar ]
21. Vincent J, Wegst U. Design and mechanical properties of insect cuticle. Arthropod Struct. Dev. 2004; 33 (3):187–199. [ PubMed ] [ Google Scholar ]
22. Durkin C, Mock T, Armbrust E. Chitin in diatoms and its association with the cell wall. Eukaryotic Cell. 2009; 8 (7):1038. [ PMC free article ] [ PubMed ] [ Google Scholar ]
23. Suzuki M, Saruwatari K, Kogure T, et al. An acidic matrix protein, Pif, is a key macromolecule for nacre formation. Science. 2009; 325 (5946):1388–1390. [ PubMed ] [ Google Scholar ]
24. Naik R, Brott L, Clarson S, Stone M. Silica-precipitating peptides isolated from a combinatorial phage display peptide library. J. nanosci. Nanotechnol. 2002; 2 (1):95–100. [ PubMed ] [ Google Scholar ] ■ The results of this study enable the interfacing of proteins and unorganic silica, which is a major material in earth and useful materials.
25. Sano K, Sasaki H, Shiba K. Specificity and biomineralization activities of Ti-binding peptide-1 (TBP-1) Langmuir. 2005; 21 (7):3090–3095. [ PubMed ] [ Google Scholar ]
26. Seker UOS, Wilson B, Sahin D, Tamerler C, Sarikaya M. Quantitative affinity of genetically engineered repeating polypeptides to inorganic surfaces. Biomacromolecules. 2008; 10 (2):250–257. [ PubMed ] [ Google Scholar ]
27. Wang WX, Dgany O, Wolf SG, et al. Aspen SP1, an exceptional thermal, protease and detergent-resistant self-assembled nano-particle. Biotechnol. Bioeng. 2006; 95 (1):161–168. [ PubMed ] [ Google Scholar ]
28. Dgany O, Gonzalez A, Sofer O, et al. The structural basis of the thermostability of SP1, a novel plant (Populus tremula) boiling stable protein. J. Biol. Chem. 2004; 279 (49):51516–51523. [ PubMed ] [ Google Scholar ]
29. Heyman A, Medalsy I, Or O, et al. Protein scaffold engineering towards tunable surface attachment. Angew. Chem. Int. Ed. 2009; 48 (49):9290–9294. [ PubMed ] [ Google Scholar ] ■ Self-assembled nanoscaffold protein for tunable interfacing between proteins and inorganic materials.
30. Wong Po Foo C, Patwardhan S, Belton D, et al. Novel nanocomposites from spider silk–silica fusion (chimeric) proteins. Proc. Natl Acad. Sci. USA. 2006; 103 (25):9428. [ PMC free article ] [ PubMed ] [ Google Scholar ]
31. Heyman A, Barak Y, Caspi J, et al. Multiple display of catalytic modules on a protein scaffold: nano-fabrication of enzyme particles. J. Biotechnol. 2007; 131 (4):433–439. [ PubMed ] [ Google Scholar ]
32. Heyman A, Medalsy I, Dgany O, Porath D, Markovich G, Shoseyov O. Float and compress: honeycomb-like array of a highly stable protein scaffold. Langmuir. 2009; 25 (9):5226–5229. [ PubMed ] [ Google Scholar ]
33. Medalsy I, Dgany O, Sowwan M, et al. SP1 protein-based nanostructures and arrays. Nano Lett. 2008; 8 (2):473–477. [ PubMed ] [ Google Scholar ]
34. Medalsy I, Klein M, Heyman A, et al. Logic implementations using a single nanoparticle–protein hybrid. Nat. Nanotechnol. 2010; 5 (6):451–457. [ PubMed ] [ Google Scholar ]
35. Gautier C, Abdoul-Aribi N, Roux C, Lopez PJ, Livage J, Coradin T. Biomimetic dual templating of silica by polysaccharide/protein assemblies. Colloids Surf. B. 2008; 65 (1):140–145. [ PubMed ] [ Google Scholar ]
36. Li J, Wu H, Liang Y, Jiang Z, Jiang Y, Zhang L. Facile fabrication of organic-inorganic hybrid beads by aminated alginate enabled gelation and biomimetic mineralization. J. Biomater Sci. Polym Ed. 2012 doi:10.1163/156856212X627351. Epub ahead of print. [ PubMed ] [ Google Scholar ]
37. Broomell C, Chase S, Laue T, Waite J. Cutting edge structural protein from the jaws of Nereis virens . Biomacromolecules. 2008; 9 (6):1669–1677. [ PubMed ] [ Google Scholar ]
38. Cribb BW, Stewart A, Huang H, et al. Insect mandibles–comparative mechanical properties and links with metal incorporation. Naturwiss. 2008; 95 (1):17–23. [ PubMed ] [ Google Scholar ]
39. Yu M, Hwang J, Deming T. Role of L-3, 4-dihydroxyphenylalanine in mussel adhesive proteins. J. Am. Chem. Soc. 1999; 121 (4):5825–5826. [ Google Scholar ]
40. Miserez A, Li Y, Waite JH, Zok F. Jumbo squid beaks: inspiration for design of robust organic composites. Acta Biomater. 2007; 3 (1):139–149. [ PubMed ] [ Google Scholar ]
41. Miserez A, Schneberk T, Sun C, Zok FW, Waite JH. The transition from stiff to compliant materials in squid beaks. Science. 2008; 319 (5871):1816–1819. [ PMC free article ] [ PubMed ] [ Google Scholar ]
42. Lee H, Rho J, Messersmith PB. Facile conjugation of biomolecules onto surfaces via mussel adhesive protein inspired coatings. Adv. Mater. 2009; 21 (4):431–434. [ PMC free article ] [ PubMed ] [ Google Scholar ]
43. Lewandowski A, Yi H, Luo X, et al. Protein assembly onto patterned microfabricated devices through enzymatic activation of fusion pro-tag. Biotechnol. Bi
Big Tits Mature Brunette
Comic Porno Ben 10
Cartoon Porn Sex Games