Mokra Taylor Drains
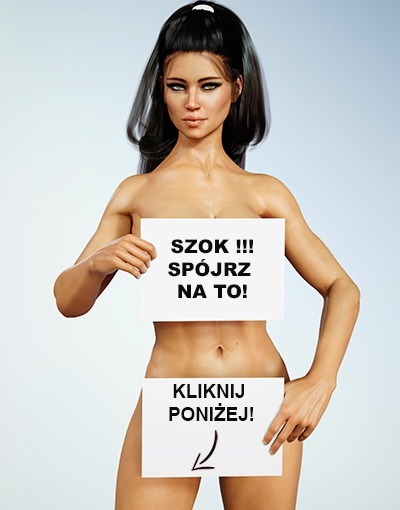
🛑 KLIKNIJ TUTAJ, ABY UZYSKAĆ WIĘCEJ INFORMACJI 👈🏻👈🏻👈🏻
Mokra Taylor Drains
Sicherheitsprüfung
Schließen
Passwort
Einblenden
العربية (Arabisch)
Čeština (Tschechisch)
Dansk (Dänisch)
Deutsch
English (Englisch)
Español (Spanisch)
Français (Französisch)
हिंदी (Hindi)
Bahasa Indonesia (Indonesisch)
Italiano (Italienisch)
日本語 (Japanisch)
한국어 (Koreanisch)
Bahasa Malaysia (Malaysisch)
Nederlands (Niederländisch)
Norsk (Norwegisch)
Polski (Polnisch)
Português (Portugiesisch)
Română (Rumänisch)
Русский (Russisch)
Svenska (Schwedisch)
ภาษาไทย (Thai)
Tagalog (Tagalog)
Türkçe (Türkisch)
Українська (Ukrainisch)
简体中文 (Chinesisch vereinfacht)
正體中文 (Chinesisch traditionell)
Sprache
Durch Klicken auf „Zustimmen & anmelden“ stimmen Sie der Nutzervereinbarung , der Datenschutzrichtlinie und der Cookie-Richtlinie von LinkedIn zu.
Bereits Mitglied bei LinkedIn? Einloggen
Download Article
Download PDF
ReadCube
EPUB
XML (NLM)
Front. Toxicol., 22 November 2021 Sec. Developmental and Reproductive Toxicology
https://doi.org/10.3389/ftox.2021.732436
Weston S. Chambers 1 , Jaida G. Hopkins 1 and Sean M. Richards 1,2 *
University of Massachusetts Amherst, United States
Faculty of Pharmacy, Sanyo-Onoda City University, Japan
Graduate School of Public Health, University of Pittsburgh, United States
The editor and reviewer's affiliations are the latest provided on their Loop research profiles and may not reflect their situation at the time of review.
Endocrine-Disrupting Compounds in Plastics and their Effects on Reproduction, Fertility, and Development
In this review article, we compiled peer-reviewed literature describing PFAS exposure and reproductive effects in animals and humans. The aim was to compare environmental occurrence and effects of the most prominent long-chain PFAS compounds and their short-chain replacements. Long-chain PFAS compounds are known to persist in the environment due to their chemical stability, and also known to bioaccumulate; hence, these compounds are being replaced globally. Indeed, PFOA and PFOS are considered long-chain “forever pollutants,” and thus the potential reproductive risk may continue for decades. Much less is known about their short-chain replacements despite the fact that they becoming more widespread in the environment. Short-chain PFAS are generally less bioaccumulative than long-chain, but they are more mobile and persistent in aquatic ecosystems. The three most prominent of these are commonly referred to as GenX, ADONA and F53B. The short-chain PFAS have similar physical and chemical properties as their predecessors; however, because they are relatively new, much less is known about the potential to disrupt reproduction. Indeed, high-quality epidemiological studies are needed to determine associations between short-chain PFAS exposure and effects on reproductive health. However, epidemiological evidence is mounting that long-chain PFAS exposure is associated with reproductive effects (i.e., decrease in fertility, reduced fetal growth and birth weight, pregnancy-induced hypertension and preeclampsia, thyroid hormone disruption during pregnancy, and preterm birth). Evidence from animal models and human cell lines indicates that short-chain PFAS similarly affect reproductive endpoints; however, epidemiological studies are scarce and inconsistent. Although short-chain PFAS have been quantified in drinking water and sediment worldwide, most of these studies did not focus on quantitation of GenX, ADONA, and F53B. There are also many other short-chain PFAS byproducts of manufacturing that have yet to be identified and studied. When sum total concentration of long- and short-chain PFAS are considered, the concentration rises by an order or magnitude or greater, as will the risk of exposure and subsequent reproductive effects.
Per- and Polyfluorinated Alkyl Substances (PFAS) are synthetic chemicals that contain at least one perfluoroalkyl group ( Birru et al., 2021 ). Perfluorooctanoic acid (PFOA) and perfluorooctane sulfonate (PFOS) are the most common PFAS detected in the environment due to their widespread use in manufacturing and chemical stability ( Huang and Jaffé, 2019 ). Both PFOS and PFOA are considered long-chain PFAS because they possess an eight-carbon backbone; their functional groups are sulfonate and carboxylate, respectively ( Tsuda, 2016 ). They possess both hydrophobic and oleophobic properties, along with other chemical characteristics making them useful in many consumer goods ( Birru et al., 2021 ). Their carbon-fluorine bonds, make them resistant to degradation and allow them to persist in the environment and bioaccumulate within living organisms ( Zeng et al., 2019 ; Neagu et al., 2021 ).
After more than 50 years of production, PFOS and PFOA were widely discovered in humans and the environment, and a subsequent phasing out of production by the 3M company began in 2000 ( Giesy and Kannan, 2001 ; Olsen et al., 2017 ). Subsequently, the United States Environmental Protection Agency procured an agreement with other major manufacturing companies to reduce long-chain PFAS emissions; other global environmental regulations for PFAS emerged as well ( Olsen et al., 2017 ). The phasing out of these compounds resulted in a decline in human blood serum concentrations of PFOS and PFOA between the years of 2000 and 2015 ( Olsen et al., 2017 ; Wang et al., 2017 ). Despite the reduction in production of long-chain PFAS, the compounds persist in the environment and in humans and are a continuing concern due to their widespread environmental persistence, distribution, potential toxicological effects, potential to bioaccumulate ( Huang and Jaffé, 2019 ).
Ingestion is considered the largest exposure route to humans (rather than dermal and inhalation). Tap water is a major PFAS exposure route where most average PFAS concentrations are in the low ng/L range ( Sinclair et al., 2020 ). Regarding PFOA and PFOS, the USEPA has issued a Health Advisory Level of 70 ppt and is in the process of making a final regulatory determination ( USEPA, 2021 ). Seafood is a significant PFAS exposure route while exposure from consumer goods, food packaging, and indoor environments are uncertain ( Sunderland et al., 2019 ).
With the phasing out of long-chain PFAS in the early 2000s, short-chain alternatives (i.e., those with carbon backbones of <7 carbons) began to take their place in industry and the environment ( Brendel et al., 2018 ). These alternatives share similar structures to long-chain PFAS (i.e., heavily fluorinated carbon chains), however, one or more alkylether group is inserted into a shorter fluoroalkyl chain. Short-chain PFAS are also products of long-chain degradation ( Li et al., 2020 ). Short-chain replacements GenX, ADONA, and F53B are widely used. GenX is a trade name for a short-chain PFAS processing technology, of which the hexafluoropropylene oxide (HFPO) dimer acid and its ammonium salt are the major constituents. ADONA (dodecafluoro-3H-4,8-dioxanonanoate) is generally used as a substitute for PFOA in fluoropolymer production ( Fromme et al., 2017 ; Munoz et al., 2019 ). Chlorinated polyfluoroalkyl ether sulfonate, or F53B, has been produced as an alternative to PFOS and has been adopted as a mist suppressant by several electroplating companies ( Du et al., 2016 ; Munoz et al., 2019 ; Shi et al., 2019 ).
Water is the major environmental sink for long- and short-chain PFAS. Bai and Son (2021) quantified concentrations of 17 PFAS in the Las Vegas and Reno watersheds (Nevada, USA), and compared them to data on aquatic PFAS concentrations worldwide. Overall, Bai and Son (2021) found that concentrations of individual PFAS ranged from non-detectable to low ppt (maximum of 74.47 ng/L) which was similar to studies and findings in watersheds of the United States ( Nakayama et al., 2007 ; Zhang et al., 2016 ), Europe ( Moller et al., 2010 ), Uganda ( Dalahmeh et al., 2018 ), China ( So et al., 2007 ; Zhao et al., 2013 ; Wang et al., 2019 ), and Australia ( Clara et al., 2009 ). In surface waters where sewage effluent is the primary input, maximum PFAS concentrations were 207.59 ng/L; groundwater contaminated with landfill leachate maximum PFAS concentrations were 5,200 ng/L ( Zhou W. et al., 2017 ). PFAS have been detected in the tissues of many wild species, including, but not limited to, bald eagles, albatrosses, polar bears, seals, dolphins, alligators, squid, and many fish species ( Giesy and Kannan, 2001 ; McCarthy et al., 2017 ).
Although atmospheric deposition is a possibility, the main source of GenX and ADONA in surface water is manufacturer’s wastewater ( Hopkins et al., 2018 ). F53B is primarily released into waterways via chrome plating industries, finding its way into nearby irrigation systems and ground water. The shorter length and alkylether substitution of short-chain PFAS contribute to a greater water solubility. GenX, when in water, loses an ammonium group, creating an anion that is the same as the HFPO-DA anion in water ( Hopkins et al., 2018 ). This anion has a precursor that travels via air, the C3 dimer acid fluoride ( Hopkins et al., 2018 ). Once the C3 dimer acid fluoride interacts with water, it creates the same anion which readily migrates to groundwater, contributing to private well contamination ( Hopkins et al., 2018 ). The limited data indicate that most short-chain PFAS are more persistent and widespread than long-chain PFAS in the aquatic environment ( Olsen et al., 2017 ; Wang et al., 2017 ; Brendel et al., 2018 ; Li et al., 2020 ). GenX chemicals are present in multiple aquatic environments including surface water, groundwater, and in drinking water and rainwater ( USEPA, 2018 ). Aside from factory wastewater effluent or points very close to industry outfalls, concentrations of GenX, ADONA, and F53B are found in surface waters in the low ng/L concentrations ( Pan et al., 2018 ; Munoz et al., 2019 ; Gebbink and van Leeuwen, 2020 ; Bai and Son, 2021 ). Both GenX and F53B were found in higher concentrations than long-chain PFAS in surface water samples collected downstream from a PFAS manufacturer ( Gebbink et al., 2017 ; Ateia et al., 2019 ). And although short-chain PFAS have been quantified in drinking water and sediment worldwide, most studies did not focus on quantitation of GenX, ADONA, and F53B and thus, data in the aquatic environment are lacking ( Ateia et al., 2019 ).
Bai and Son (2021) found that short-chain PFAS were more likely to partition in the water column, while long-chain PFAS most likely to partition in sediment. This is similar to the finding of Zhao et al. (2016) who estimated that 88.8% of total PFAS in the water column are short-chain. These estimations correspond to what would be expected based on the greater water solubility of short-chain PFAS.
It is important to note that the studies that concluded “relatively low” concentrations of PFAS in the water column or sediment are often describing the concentration of individual molecules. While environmental regulations limit concentrations of individual PFAS, the sum of the thousands of PFAS need to be considered for estimations of exposure and effects. Indeed, even if toxicity is considered to be simply additive, estimations of risk must include total PFAS exposure.
Routes of human exposure to PFAS include food, drinking water, house dust, ambient and indoor air, and consumer products ( Olsen et al., 2017 ). Examples of consumer products that have utilized PFAS in the past include fabrics, carpet, grease-proof food-contact paper, nonstick cookware, paints, and cosmetics ( Kjeldsen and Bonefeld-Jørgensen, 2013 ; Olsen et al., 2017 ).
For populations close to sources of contaminated water sources, drinking water is the dominant exposure pathway ( Vestergren and Cousins, 2009 ). This was illustrated by Fromme et al. (2017) ; blood plasma samples were tested for the presence of PFOS, PFOA, and ADONA in humans living within an 80 km radius of a former PFOA production plant, where ADONA was being used as a replacement emulsifier. Drinking water was the known exposure route. The 95th percentile concentrations in blood plasma were 13.5 μg/L and 85.5 μg/L for PFOS and PFOA, respectively. Blood plasma concentrations of ADONA were just above detection levels (0.2 μg/L), indicating that health risks were not likely ( Fromme et al., 2017 ).
Food is also a major source of exposure, PFAS have been detected in fish fillets, blood serum of beef cattle, fruits and vegetables, bread, and milk ( Vestergren and Cousins, 2009 ). Human breast milk is a source of infant exposure to PFAS, and these compounds have been detected in samples in the United States, Asia, and Europe ( Tao et al., 2008 ). PFAS have also been found in human umbilical cord blood samples, with one study detecting trace amounts in 100% of tested samples ( Apelberg et al., 2007 ). These findings, indicating an exposure pathway in utero , are concerning due to the high vulnerability to toxicants during fetal development ( Bach et al., 2014 ).
PFOS and PFOA are readily absorbed in the gastrointestinal tract, excreted in urine and feces, and do not undergo metabolism. Estimated human half-lives for PFOS and PFOA are approximately 5 years and 2–4 years, respectively (EFSA, 2018). PFAS bind to blood serum proteins, allowing them to travel through the circulatory system and subsequently accumulate in most organs in the human body, particularly in the kidneys, liver, spleen, brain, and testicles ( Jensen and Leffers, 2008 ; Wang et al., 2021 ). Elimination of PFAS from the body is done via renal clearance. Clearance varies widely by species. In humans it is a relatively slow process with half-lives of 3.8 years for PFOA and 5.4 years for PFOS ( Behr et al., 2018 ). Much shorter half-lives are observed in other animals, with male mice, rabbits, chickens, and cynomolgus monkeys having half-lives of 12, 0.23, 4.6, and 30 days, respectively ( Vestergren and Cousins, 2009 ). For water-breathing organisms, the high aqueous solubility allows for easier elimination of the compounds through the gills ( Kelly et al., 2009 ).
Another reason for the phasing out of long-chain PFAS is the potential of these compounds to bioaccumulate ( Olsen et al., 2017 ). In Lake Ontario, the concentrations of long-chain PFAS were higher within the biota and sediment than the water, giving a bioaccumulation factor between lake trout and water of 3.4 × 10 4 L/kg ( Houde et al., 2008 ). Zooplankton contained 195 ng/g, sediment contained 187 ng/g, and the water contained 2.2 ng/L ( Houde et al., 2008 ). Some predatory species have been observed to contain higher levels of PFAS than in their diets, indicating that PFAS can biomagnify in higher trophic levels ( Giesy and Kannan, 2001 ). Biomagnification is seen only in non-aquatic predators, such as seals and polar bears, most likely due to the proteinophilic nature of PFAS, relatively high aqueous solubility of PFAS, and the low volatility ( Kelly et al., 2009 ).
Because of the increased water solubility of short-chain PFAS, most studies indicated a lower bioaccumulation potential. Indeed, short-chain PFAS are eliminated from the body quicker, and therefore have less potential to bioaccumulate ( Gomis et al., 2018 ). Carbon-chain length is generally inversely proportional with elimination rates; this trend follows for humans as well ( Lau, 2012 ). For example, a four carbon PFAS, perfluorobutanoate (PFBA), had a measured half-life of 3 days in humans, which is a stark difference from the years-long half-lives of PFOS and PFOA ( Lau, 2012 ). While short-chain PFAS may not remain in biota for extended periods of time, this does not necessarily indicate that they have less potential toxicity than long-chain PFAS ( Gomis et al., 2018 ). Short-chain PFAS are still very persistent in the environment, indicating that there is po
Lizocipki na dworze
Ostry seks z Olgą
Ładuje spermę na jej twarz