Lristen Archives
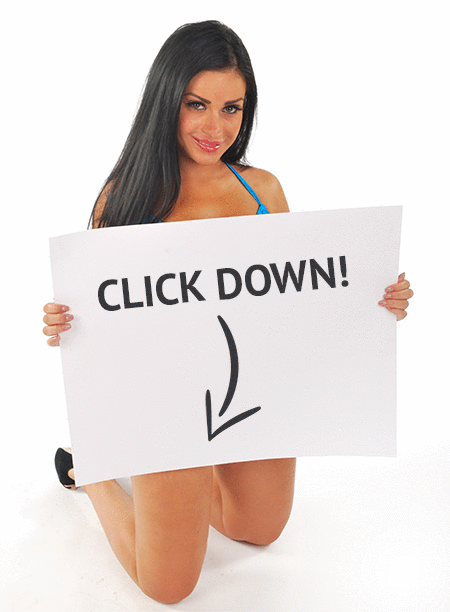
⚡ ALL INFORMATION CLICK HERE 👈🏻👈🏻👈🏻
Lristen Archives
John C. Fiala , Marcia Feinberg , Viktor Popov and Kristen M. Harris
Journal of Neuroscience 1 November 1998, 18 (21) 8900-8911; DOI: https://doi.org/10.1523/JNEUROSCI.18-21-08900.1998
1 Division of Neuroscience in the Department of Neurology, Children’s Hospital, Boston Massachusetts, and
1 Division of Neuroscience in the Department of Neurology, Children’s Hospital, Boston Massachusetts, and
2 Institute of Cell Biophysics, Russian Academy of Sciences, Pushchino, Moscow Region, 142292, Russia
1 Division of Neuroscience in the Department of Neurology, Children’s Hospital, Boston Massachusetts, and
Berry M , Hollingworth T , Flinn RM , Anderson EM ( 1972 ) The growth of Purkinje cell dendrites of the rat –a quantitative study. J Anat 111 : 491 – 493 .
Boyer C , Schikorski T , Stevens CF ( 1998 ) Comparison of hippocampal dendritic spines in culture and in brain. J Neurosci 18 : 5294 – 5300 .
Collin C , Miyaguchi K , Segal M ( 1997 ) Dendritic spine density and LTP induction in cultured hippocampal slices. J Neurophysiol 77 : 1614 – 1623 .
Cooper MW , Smith SJ ( 1992 ) A real-time analysis of growth cone-target cell interactions during the formation of stable contacts between hippocampal neurons in culture. J Neurobiol 23 : 814 – 828 .
Cotman C , Taylor D , Lynch G ( 1973 ) Ultrastructural changes in synapses in the dentate gyrus of the rat during development. Brain Res 63 : 205 – 213 .
Dailey ME , Smith SJ ( 1996 ) The dynamics of dendritic structure in developing hippocampal slices. J Neurosci 16 : 2983 – 2994 .
Davenport RW , Dou P , Mills LR , Kater SB ( 1996 ) Distinct calcium signaling within neuronal growth cones and filopodia. J Neurobiol 31 : 1 – 15 .
Difiglia M , Pasik P , Pasik T ( 1980 ) Early postnatal development of the monkey neostriatum: a Golgi and ultrastructural study. J Comp Neurol 190 : 303 – 331 .
Dvergsten CL , Hull CD , Levine MS , Adinolfi AM , Buchwald NA ( 1986 ) Postnatal differentiation and growth of cat entopeduncular neurons. A transient spiny period associated with branch elongation. Brain Res 389 : 239 – 251 .
Fisher GW , Conrad PA , DeBiasio RL , Taylor DL ( 1988 ) Centripetal transport of cytoplasm, actin, and the cell surface in lamellipodia of fibroblasts. Cell Motil Cytoskeleton 11 : 235 – 247 .
Galofre E , Ferrer I , Fabregues I , Lopez-Tejero D ( 1987 ) Effects of prenatal ethanol exposure on dendritic spines of layer V pyramidal neurons in the somatosensory cortex of the rat. J Neurol Sci 81 : 185 – 195 .
Harris KM , Kater SB ( 1994 ) Dendritic spines: cellular specializations imparting both stability and flexibility to synaptic function. Annu Rev Neurosci 17 : 341 – 371 .
Harris KM , Jensen FE , Tsao B ( 1992 ) Three-dimensional structure of dendritic spines and synapses in rat hippocampus (CA1) at postnatal day 15 and adult ages: implications for the maturation of synaptic physiology and long-term potentiation. J Neurosci 12 : 2685 – 2705 .
Juraska JM , Fifkova E ( 1979 ) An electron microscope study of the early postnatal development of the visual cortex of the hooded rat. J Comp Neurol 183 : 257 – 268 .
Kossel AH , Williams CV , Schweizer M , Kater SB ( 1997 ) Afferent innervation influences the development of dendritic branches and spines via both activity-dependent and non-activity-dependent mechanisms. J Neurosci 17 : 6314 – 6324 .
Landis DMD ( 1987 ) Initial junctions between developing parallel fibers and Purkinje cells are different from mature synaptic junctions. J Comp Neurol 260 : 513 – 525 .
Linke R , Soriano E , Frotscher M ( 1994 ) Transient dendritic appendages on differentiating septohippocampal neurons are not the sites of synaptogenesis. Dev Brain Res 83 : 67 – 78 .
Lund JS , Boothe RG , Lund RD ( 1977 ) Development of neurons in the visual cortex (area 17) of the monkey ( Macaca nemestrina ): a Golgi study from fetal day 127 to postnatal maturity. J Comp Neurol 176 : 149 – 188 .
Marin-Padilla M ( 1972 ) Structural abnormalities of the cerebral cortex in human chromosomal aberrations: a Golgi study. Brain Res 44 : 625 – 629 .
Markham JA , Fifkova E ( 1986 ) Actin filament organization within dendrites and dendritic spines during development. Dev Brain Res 27 : 263 – 269 .
Mates SL , Lund JS ( 1983 ) Spine formation and maturation of type 1 synapses on spiny stellate neurons in primate visual cortex. J Comp Neurol 221 : 91 – 97 .
Miller M , Peters A ( 1981 ) Maturation of rat visual cortex. II. A combined Golgi-electron microscope study of pyramidal cells. J Comp Neurol 203 : 555 – 573 .
Morest DK ( 1969a ) The differentiation of cerebral dendrites: a study of the post-migratory neuroblast in the medial nucleus of the trapezoid body. Z Anat Entwicklungsgesch 128 : 271 – 289 .
Morest DK ( 1969b ) The growth of dendrites in the mammalian brain. Z Anat Entwicklungsgesch 128 : 290 – 317 .
O’Rourke NA , Cline HT , Fraser SE ( 1994 ) Rapid remodeling of retinal arbors in the tectum with and without blockade of synaptic transmission. Neuron 12 : 921 – 934 .
Papa M , Bundman MC , Greenberger V , Segal M ( 1995 ) Morphological analysis of dendritic spine development in primary cultures of hippocampal neurons. J Neurosci 15 : 1 – 11 .
Pappas GD , Fox GQ , Masurovsky EB , Peterson ER , Crain SM ( 1975 ) Neuronal growth cone relationships and their role in synaptogenesis in the mammalian central nervous system. in Advances in Neurology, Vol 12 , ed Kreutzberg GW ( Raven , New York ), pp 163 – 180 .
Peters A , Kaiserman-Abramof IR ( 1970 ) The small pyramidal neuron of the rat cerebral cortex: the perikaryon, dendrites and spines. J Anat 127 : 321 – 356 .
Pfenninger KH , Rees RP ( 1976 ) From growth cone to the synapse: properties of membranes involved in synapse formation. in Neuronal recognition , ed Barondes SH ( Plenum , New York ), pp 131 – 178 .
Pokorny J , Yamamoto T ( 1981 ) Postnatal ontogenesis of hippocampal CA1 area in rats. II. Development of ultrastructure in stratum lacunosum and moleculare. Brain Res Bull 7 : 121 – 130 .
Purpura DP ( 1975 ) Dendritic differentiation in human cerebral cortex: Normal and aberrant development patterns. in Advances in neurology, Vol 12 , ed Kreutzberg GW ( Raven , New York ), pp 91 – 116 .
Saito Y , Song W-J , Murakami F ( 1997 ) Preferential termination of corticorubral axons on spine-like dendritic protrusions in developing cat. J Neurosci 17 : 8792 – 8803 .
Schwartz IR , Pappas GD , Purpura DP ( 1968 ) Fine structure of neurons and synapses in the feline hippocampus during postnatal ontogenesis. Exp Neurol 22 : 394 – 407 .
Schwartzkroin PA , Kunkel DD , Mathers LH ( 1982 ) Development of rabbit hippocampus: anatomy. Dev Brain Res 2 : 453 – 468 .
Sheetz MP , Wayne DB , Pearlman AL ( 1992 ) Extension of filopodia by motor-dependent actin assembly. Cell Motil Cytoskeleton 22 : 160 – 169 .
Shepherd GMG, Harris KM (1998) Three-dimensional structure and composition of CA3- > CA1 axons in rat hippocampal slices: implications for presynaptic connectivity and compartmentalization. J Neurosci, in press.
Smith CL ( 1994 ) Cytoskeletal movements and substrate interactions during initiation of neurite outgrowth by sympathetic neurons in vitro . J Neurosci 14 : 384 – 398 .
Smith SJ ( 1988 ) Neuronal cytomechanics: the actin based motility of growth cones. Science 242 : 708 – 715 .
Spacek J , Harris KM ( 1997 ) Three-dimensional organization of smooth endoplasmic reticulum in hippocampal CA1 dendrites and dendritic spines of the immature and mature rat. J Neurosci 17 : 190 – 203 .
Steward O , Falk PM ( 1991 ) Selective localization of polyribosomes beneath developing synapses: A quantitative analysis of the relationships between polyribosomes and developing synapses in the hippocampus and dentate gyrus. J Comp Neurol 314 : 545 – 557 .
Stoltenburg-Didinger G , Spohr HL ( 1983 ) Fetal alcohol syndrome and mental retardation: spine distribution of pyramidal cells in prenatal alcohol-exposed rat cerebral cortex: a Golgi study. Brain Res 313 : 119 – 123 .
Ulfhake B , Cullheim S ( 1988 ) Postnatal development of cat hind limb motoneurons. II: In vivo morphology of dendritic growth cones and the maturation of dendrite morphology. J Comp Neurol 278 : 88 – 102 .
Vaughn JE ( 1989 ) Fine structure of synaptogenesis in the vertebrate central nervous system. Synapse 3 : 255 – 285 .
Wisniewski KE , Segan SM , Miezejeski CM , Sersen EA , Rudelli RD ( 1991 ) The Fra(X) syndrome: neurological, electrophysiological, and neuropathological abnormalities. Am J Med Genet 38 : 476 – 480 .
Wong ROL , Yamawaki RM , Shatz CL ( 1992 ) Synaptic contacts and the transient dendritic spines of developing retinal ganglion cells. Eur J Neurosci 4 : 1387 – 1397 .
Ziv NE , Smith SJ ( 1996 ) Evidence for a role of dendritic filopodia in synaptogenesis and spine formation. Neuron 17 : 91 – 102 .
Synaptogenesis Via Dendritic Filopodia in Developing Hippocampal Area CA1
John C. Fiala , Marcia Feinberg , Viktor Popov , Kristen M. Harris
Journal of Neuroscience 1 November 1998, 18 (21) 8900-8911; DOI: 10.1523/JNEUROSCI.18-21-08900.1998
Share This Article:
Copy
No eLetters have been published for this article.
Enter multiple addresses on separate lines or separate them with commas.
Message Subject
(Your Name) has forwarded a page to you from Journal of Neuroscience
Message Body
(Your Name) thought you would be interested in this article in Journal of Neuroscience.
This question is for testing whether or not you are a human visitor and to prevent automated spam submissions.
Synaptogenesis Via Dendritic Filopodia in Developing Hippocampal Area CA1
John C. Fiala , Marcia Feinberg , Viktor Popov , Kristen M. Harris
Journal of Neuroscience 1 November 1998, 18 (21) 8900-8911; DOI: 10.1523/JNEUROSCI.18-21-08900.1998
To determine the role of dendritic filopodia in the genesis of excitatory synaptic contacts and dendritic spines in hippocampal area CA1, serial section electron microscopy and three-dimensional analysis of 16 volumes of neuropil from nine male rat pups, aged postnatal day 1 (P1) through P12, were performed. The analysis revealed that numerous dendritic filopodia formed asymmetric synaptic contacts with axons and with filopodia extending from axons, especially during the first postnatal week. At P1, 22 ± 5.5% of synapses occurred on dendritic filopodia, with 19 ± 5.9% on filopodia at P4, 20 ± 8.0% at P6, decreasing to 7.2 ± 4.7% at P12 ( p < 0.02). Synapses were found at the base and along the entire length of filopodia, with many filopodia exhibiting multiple synaptic contacts. In all, 162 completely traceable dendritic filopodia received 255 asymmetric synaptic contacts. These synapses were found at all parts of filopodia with equal frequency, usually occurring on fusiform swellings of the diameter. Most synaptic contacts (53 ± 11%) occurred directly on dendritic shafts during the first postnatal week. A smaller but still substantial portion (32 ± 12%) of synapses were on shafts at P12 ( p < 0.036). There was a highly significant ( p < 0.0002) increase in the proportion of dendritic spine synapses with age, rising from just 4.9 ± 4.3% at P1 to 37 ± 14% at P12. The concurrence of primarily shaft and filopodial synapses in the first postnatal week suggests that filopodia recruit shaft synapses that later give rise to spines through a process of outgrowth.
Most excitatory synapses in adult brain are located on the bulbous heads of dendritic spines ( Harris and Kater, 1994 ). During development, before the expression of spines, cortical dendrites exhibit longer, thinner processes, often without a bulbous head ( Purpura, 1975 ). These dendritic filopodia disappear, and spines appear as the cortex matures. This transition can be disrupted by developmental abnormalities that induce mental retardation, such as fetal alcohol syndrome ( Purpura, 1975 ; Stoltenburg-Didinger and Spohr, 1983 ; Galofre et al., 1987 ). Dendrites from mentally retarded adults often appear to be covered with dendritic filopodia instead of spines ( Marin-Padilla, 1972 ; Wisniewski et al., 1991 ). The significance of these findings is not entirely clear, partly because the role of dendritic filopodia in synapse and spine formation is uncertain.
Several recent in vitro studies have shown that filopodia are active along the lengths of dendrites during postnatal synaptogenesis in hippocampal area CA1. Confocal microscopy of dissociated hippocampal neurons from embryonic or neonatal rats revealed long, headless dendritic protrusions during the initial 1–2 weeks in culture ( Papa et al., 1995 ; Ziv and Smith, 1996 ). These filopodia were remarkably transient structures, extending and retracting in <10 min in some cases. Although some filopodia made apparent synaptic contacts with axons ( Ziv and Smith, 1996 ), ultrastructural examination found that the majority of filopodia at 1 week in culture had no synaptic contacts ( Papa et al., 1995 ). Over a period of 4 weeks in culture, there was a transition from relatively sparse, yet dynamic filopodial protrusions, to a dense distribution of stable, adult-like spines. Similar results were obtained from area CA1 in hippocampal slice cultures ( Dailey and Smith, 1996 ; Collin et al., 1997 ).
The appearance of filopodia before the formation of spines, and the fact that some filopodia retract into a more stable, spine-like shape has lead to the hypothesis that most spines form directly from filopodia ( Ziv and Smith, 1996 ). This hypothesis posits that filopodia initiate synaptic contacts that mature into spine synapses. In older cultures, rapid extension of stable spines directly from the dendrite shafts was observed without the prior appearance of dynamic filopodia ( Dailey and Smith, 1996 ). This suggests that spines can arise from shaft synapses and is consistent with ultrastructural data showing that synapses occur primarily on dendritic shafts and stubby spines before postnatal day 15 (P15), with a subsequent transition to most synapses on longer thin spines in the young adult rat ( Schwartz et al., 1968 ; Cotman et al., 1973 ; Miller and Peters, 1981 ; Porkorny and Yamamoto, 1981 ; Schwartzkroin et al., 1982 ; Steward and Falk, 1991 ; Harris et al., 1992 ).
To determine whether dendritic filopodia make synaptic contacts and whether filopodia could be involved in the production of shaft synapses, we conducted a serial electron microscopy analysis of in vivo development of synaptic contacts in area CA1. Volumetric analyses were used to identify the three-dimensional structures (dendrites, spines, and filopodia) on which synapses occurred. The results show that filopodial synapses develop concomitantly with an abundance of shaft synapses, before spine formation. The data support a role for filopodia in the genesis of shaft synapses, which later develop into dendritic spines.
Animals. Nine male rat pups of the Long–Evans strain at four different ages were used (Table 1 ). Three animals were from P1, and two each were from P4, P6, and P12. Our procedures follow National Institutes of Health guidelines and undergo yearly review by the Animal Care and Use Committee at Children’s Hospital.
Location, volume, and number of synapses for each set of serial section electron micrographs. R numbers are individual rat numbers for a total of nine rats; letters a, b, and c are the specific series identifiers
Tissue preparation and microscopy. Animals were perfused through the heart under deep (80 mg/kg) pentobarbital anesthesia with 2.5% glutaraldehyde, 2% paraformaldehyde, 2 m m CaCl 2 , and 4 m m MgCl 2 in 0.1 m cacodylate buffer. Whole brains were removed after 1 hr and post-fixed overnight in the same fixative. The next day the brain was rinsed in buffer and sliced into 300 μm slabs, from which the hippocampus with dentate gyrus was dissected.
Routine processing of the hippocampi for electron microscopy (EM) was accelerated using the PELCO 3450 laboratory microwave processor (Ted Pella Inc., Redding, CA). A slab of hippocampus was placed in an individual microcentrifuge tube with 1% osmium and 1.5% potassium ferrocyanide in 0.1 m cacodylate buffer solution, cooled in an ice bath to <15°C, then microwaved for 2.5 min at 37°C. After several buffer rinses, a second stage osmium solution (1% osmium in 0.1 m cacodylate buffer) was added, cooled to <15°C, and then microwaved again for 2.5 min at 37°C. The tissue was rinsed several times in buffer followed by two brief water rinses and then a 1% aqueous uranyl acetate was added for en bloc staining. This solution was also cooled to <15°C and then microwaved for 2.5 min at 37°C, followed by two brief water rinses and then dehydrated through graded acetones beginning with 50%, then 70, 90, and 100% exchanges in the microwave at 45°C, 40 sec each exchange. The dehydrated tissue was infiltrated in the microwave using acetone mixed with 1:1 Epon and Spurr’s resin mixtures for 15 min at 50°C. This was followed by two changes of fresh 100% Epon/Spurr’s for 15 min each at 50°C. For final polymerization and curing the tissue was transferred to BEEM embedding capsules. Some tissue was cured in the microwave and others were cured in a 60°C conventional oven for 48 hr.
The cured blocks were cut with a diamond Histo knife on a Reichart Ultracut S (Leica, Allendale, NJ) ultramicrotome to obtain a full-face section (1 μm thickness) of the hippocampus and dentate gyrus. These were stained with 1% toluidine blue and examined under light microscopy. A trapezoid, 30–115 μm in height and 50–115 μm base width, was cut from the apical dendritic field of area CA1 using a Diatome square-shaped trim tool (Electron Microscopy Sciences, Fort Washington, PA). Table 1 gives the location of each trapezoid, illustrating how both proximal and distal portions of the apical field were sampled at each age.
Serial sections were made from the trimmed blocks with silver to platinum coloring (nominally 70 nm). The number of sections varied from 52 to 135 for each of the 16 series. Series were mounted on Synaptek pioloform-coated slot grids (Ted Pella Inc.) and stained with saturated ethanolic uranyl acetate, followed by Reynold’s lead citrate, each for 5 min. Each grid was then loaded into a grid cassette (Advanced Microscopy Techniques, Danvers, MA) and stored in numbered gelatin capsules (Ernest Fullam Inc., Latham, NY). The grid cassettes were mounted in rotating stages to obtain consistent orientation of sections on adjacent grids during photography at a JEOL (Peabody, MA) 1200EX electron microscope. Each series was photographed at 6000× magnification along with a corresponding calibration grid (0.463 μm per square, Ernest Fullam Inc.) and printed at 16000× magnification on 8.5 × 11 inch paper.
Analysis. A volume of tissue was analyzed from the middle of each series of micrographs so that neuronal structures (synapses, axons, dendrites, spines, and filopodia) could be traced through the series and identified by three-dimensional (3D) structure (Table 1 ). Each micrograph in the volume was systematically scanned for synapses. Asymmetric synapses
Playforceone Game
Free Uncensored Hentai Manga
Abby Elliot Nude