Галерея 3496121
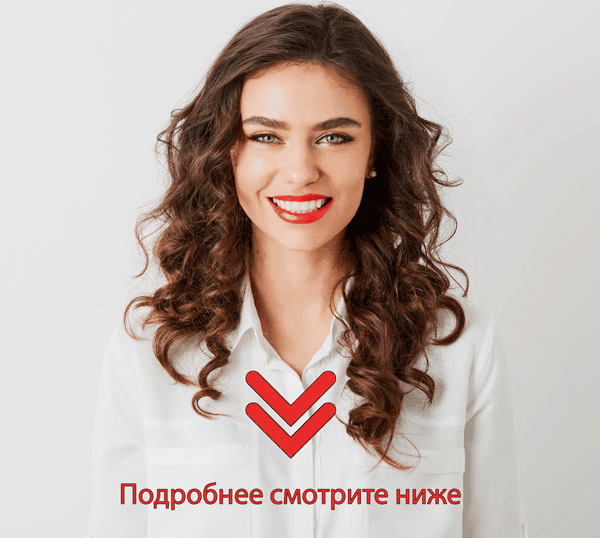
⚡ ПОДРОБНЕЕ ЖМИТЕ ЗДЕСЬ 👈🏻👈🏻👈🏻
Галерея 3496121
Dashboard
Publications
Account settings
Log out
Journal List
Breast Cancer Res
v.14(1); 2012
PMC3496121
Create a new collection
Add to an existing collection
Unable to load your collection due to an error
Please try again
Breast Cancer Res. 2012; 14(1): R6.
Published online 2012 Jan 7. doi: 10.1186/bcr3087
Find articles by Danielle L Peacock
1 Center for Cancer Research and the Department of Pathology and Laboratory Medicine, The University of Tennessee Health Science Center, Cancer Research Building, 19 South Manassas Street, Memphis, TN 38163, USA
Luciana P Schwab: ude.cshtu@bawhcsl ; Danielle L Peacock: ufde.cshtu@3cocaepd ; Debeshi Majumdar: moc.oohay@5002_ihsebed ; Jesse F Ingels: ude.cshtu@slegnij ; Laura C Jensen: ude.cshtu@3nesnejl ; Keisha D Smith: ude.cshtu@581timsk ; Richard C Cushing: ude.cshtu@gnihsucr ; Tiffany N Seagroves: ude.cshtu@1orgaest
Received 2011 Jun 14; Revised 2011 Nov 29; Accepted 2012 Jan 7.
Copyright ©2012 Schwab et al.; licensee BioMed Central Ltd.
This is an open access article distributed under the terms of the Creative Commons Attribution License ( http://creativecommons.org/licenses/by/2.0 ), which permits unrestricted use, distribution, and reproduction in any medium, provided the original work is properly cited.
Additional file 1 Table S1 Primers and Roche Universal Probe Library (UPL) 6-carboxyfluorescein-labeled probes utilized in quantitative RT-PCR assays . Table S2 Primary antibody source and dilution factors utilized in Western blotting, immunohistochemistry (IHC), immunofluorescence (IF) and fluorescence-activated cell sorting (FACS) . Anti-α-SMA, anti-α-smooth muscle actin; anti-mouse CD133-PE, anti-mouse CD133-phycoerythrin; anti-mouse ERα, anti-mouse estrogen receptor α; anti-mouse CD24-FITC, anti-mouse CD24-fluorescein isothiocyanate; HRP, horseradish peroxidase; SA-APC, streptavidin-allophycocyanin. Table S3 Frequency of tumors in recipient mice at day 62 after limiting dilution transplantation . ELDA, Extreme Limiting Dilution Analysis; HIF-1α, hypoxia-inducible factor 1α; KO, knockout; MTEC, mammary tumor epithelial cell; TIC, tumor-initiating cell; WT, wild type. Table S4 Frequency of tumors in recipient mice at day 112 after limiting dilution transplantation . ELDA, Extreme Limiting Dilution Analysis; HIF-1α, hypoxia-inducible factor 1α; KO, knockout; MTEC, mammary tumor epithelial cell; TIC, tumor-initiating cell; WT, wild type. Table S5 Summary of the percentage and total number of recipients bearing small tumors at day 112 posttransplant . KO, knockout; WT, wild type. Table S6 Frequency of tumors in recipient mice at day 244 after limiting dilution transplantation . ELDA, Extreme Limiting Dilution Analysis; HIF-1α, hypoxia-inducible factor 1α; KO, knockout; MTEC, mammary tumor epithelial cell; TIC, tumor-initiating cell; WT, wild type. Table S7 Summary of the percentage and total number of recipients bearing small tumors at day 244 posttransplant . KO, knockout; WT, wild type.
GUID: 38CB410E-8DA4-4EC4-A497-3512F7EE3661
Additional file 2 Figure S1 HIF-1α expression increase during tumor progression in the MMTV-PyMT mouse model and confirmation of HIF-1α deletion . HS-WCE protein extracts were prepared from two individual transgenic MMTV-PyMT female mice (FVB/Nj strain) between 6 and 9 weeks of age. HS-WCE, high-salt whole-cell extract; MMTV-PyMT, mouse mammary tumor virus polyoma virus middle T. By palpation, each mouse had at least one mammary gland with hyperplastic (HP) (palpable as grainy), early-carcinoma (EC) (< 250 mm 3 ) or late-carcinoma (LC) (> 500 mm 3 ) lesions, which was expected because tumor progression among glands is asynchronous in this model. Two independent glands (one from each mouse) per stage were utilized to prepare HS-WCEs for Western blotting. As shown, HIF-1α expression generally increased during progression and was most abundant in late-stage carcinomas. Included as controls were HS-WCEs prepared from wild-type (WT) and knockout (KO) mammary tumor epithelial cells (MTECs) grown to 80% confluence, followed by hypoxia exposure for 6 hours at 0.5% O 2 . A cross-reactive material (CRM) band at about 76 kDa was detectable when lot E2 of NB 100-479 of the anti-mouse HIF-1α primary antibody (Novus Biologicals, Littleton, CO, USA) was used. KO cells were transduced twice with adenovirus-Cre in monolayer culture at 80 to 100 plaque-forming units/cell to achieve > 99% deletion efficiency as determined by qRT-PCR and Western blot analysis. Figure S2 HIF-1α expression increases in response to EGF treatment at normoxia, and EGF prolongs HIF-1α stabilization at hypoxia . Wild-type (WT) mammary tumor epithelial cells (MTECs) were either cultured in complete growth medium (containing 5% fetal bovine serum, FBS + 5 μg/ml insulin + 10 ng/ml epidermal growth factor, EGF) or in medium supplemented only with 2% FBS for at least five passages prior to replating to test the effect of EGF treatment on HIF-1α expression. Cells grown to 80% confluence were incubated at hypoxia for the number of hours indicated (3, 6 or 24). All cells were exposed to hypoxia beginning 24 hours prior to harvest (the t = 0 time point) such that cells at the t = 0 time point (normoxic control) were harvested at the same time as the t = 24 hours hypoxia time point. Cells were exposed to hypoxia for the indicated number of hours prior to removal from culture and the immediate preparation of high-salt enriched whole-cell extracts. HIF-1α was detected using lot E2 of NB 100-479 (Novus Biologicals, Littleton, CO, USA). Figure S3 Histology of HIF-1α WT and KO end-stage PyMT tumors . H & E-stained sections of end-stage tumors (> 750 mm 3 ) derived from wild-type (WT) or knockout (KO) cells are shown. HIF-1α, hypoxia-inducible factor 1α; PyMT, polyoma virus middle T. The WT tumors typically contained more extensive areas of necrosis than the KO tumors. One representative tumor per genotype was imaged using 5 ×, 20 × and 63 × lens objectives of a Leica DM6000 upright microscope (Leica Microsystems, Buffalo Grove, IL, USA) with a SPOT camera (SPOT Imaging Solutions, Sterling Heights, MI, USA) for final original magnifications of ×50, ×200 or ×630, respectively. Scale bar = 50 μm. Digitally scanned whole slides of H & E-stained WT and KO tumor sections were captured using the Aperio ScanScope system (Aperio, Vista, CA, USA). Whole-slide images have been posted to a public database, which may be accessed using instructions provided in the Additional methods. Figure S4 . Ki67 and activated caspase 3 immunostaining in end-stage PyMT tumors . Paraffin-embedded, formalin-fixed tissue sections from wild-type (WT) and knockout (KO) tumors (> 500 to 750 mm 3 ) were immunostained with either Ki67 or activated caspase 3 antibodies, and immunoreactive complexes were detected using the VECTASTAIN Elite ABC Kit (Vector Laboratories, Burlingame, CA, USA) and developed with ImmPACT DAB substrate (diaminobenzidine; Vector Laboratories), followed by counterstaining with Harris hematoxylin. PyMT, polyoma virus middle T. Scale bar = 100 μm. Figure S5 Expression of p63 and ERα in WT and KO end-stage PyMT tumors . Representative results of p63 and estrogen receptor α (ERα) immunostaining in wild-type (WT) and knockout (KO) tumors ( n = 4/genotype), along with expression observed in normal virgin mammary glands, which served as the positive control ( n = 2 FVB strain mice about 8 weeks of age). HIF-1α, hypoxia-inducible factor 1α. Original magnification, ×200; scale bar = 50 μm. Black arrows point to examples of positive cells. No obvious changes in p63 expression or localization were detected between WT and KO tumors, whereas p63 was located basally within the virgin mammary ductal tree, as expected. No immunoreactivity for ERα was detected in end-stage WT or KO tumors (which were derived from late-stage carcinomas of the MMTV-PyMT transgenic mouse), whereas almost all ductal epithelial cells in the virgin normal mammary gland expressed ERα as previously reported [ 77 ]. Figure S6 Overview of the mean fold enrichment in SFE in WT cells compared to KO cells . (A) to (C) Bar graphs represent the mean fold enrichment of sphere formation efficiency (SFE) ± SEM for each generation (three biological replicates per genotype per generation in (A) and (B)) or for specific genotype-oxygen tension comparisons (C). Adeno-Bgal, adenovirus β-galactosidase; DF, double floxed ; HIF, hypoxia-inducible factor 1α; KO, knockout; PyMT+, polyoma virus middle T-positive; WT, wild type. (C) The mean fold change in SFE observed for specific comparisons between genotype and oxygen tension is presented (nor, normoxia; hyp, hypoxia) (wild-type normoxia (WT nor) to knockout (KO) nor, n = 4; WT hypoxia (WT hyp) to KO hyp, n = 3; WT nor to WT hyp, n = 5; KO nor to KO hyp, n = 3). The SFE is a percentage and was determined as follows: [(number of cells capable of forming spheres)/(total number of single cells plated) × 100]. Figure S7 HIF-1α expression in tumorspheres cultured acutely or chronically at hypoxia . Wild type (WT) or knockout (KO) tumorspheres were originally derived from single cells plated into ultralow adhesion dishes and cultured at normoxia. HIF-1α, hypoxia-inducible factor 1α. A subset of WT and KO tumorspheres (80 to 100 μm in size) were then transferred from normoxic to hypoxic culture (0.5% O 2 ) for the duration of incubation, which was either acute (6-hour exposure) or chronic (> 4 days' exposure). Spheres were then isolated, high-salt whole-cell extract (HS-WCE) was prepared and HIF-1α expression levels were evaluated by Western blot analysis (input of 1 μg HS-WCE/lane) using lot M1 of Novus 100-479 (Novus Biologicals, Littleton, CO, USA), which produced more cross-reactive material (CRM) bands than previously observed with lot E2. Figure S8 Gating strategy for sorting CD133 hi versus CD133 neg populations from mammary tumors originating in PyMT+ transgenic females . After being stained, cells resuspended in flow buffer were subjected to flow sorting on a FACSAria cell sorter (BD Biosciences, San Diego, CA, USA) at the University of Tennessee Health Science Center Flow Cytometry and Sorting Core. Forward scatter (FSC) and side scatter (SSC) gates were set to exclude particulate debris, followed by gating for singlets based on FSC area (FSC-A) and FSC height (FSC-H). Singlet events were determined to be viable by gating against cells that stained positive with SYTOX Blue (Molecular Probes/BD Biosciences, Eugene, OR, USA) or 7-AAD (BD Pharmingen, San Diego, CA, USA). Lin neg live singlets were selectively gated based on the absence of expression of the mouse lineage marker panel (BD Biosciences) that was supplemented with CD31 (BD Biosciences) to exclude endothelial cells. The gating to enrich for CD133-PE hi cells was manually set based on the isotype matched control antibody. PyMT+, polyoma virus middle T-positive; APC, allophycocyanin; IgG, immunoglobulin G; Lin, hematopoietic lineage panel; PE, phycoerythrin. Figure S9 Comparison of WT and KO tumor-initiating potential at day 62 posttransplant . The percentage of FVB recipients (tumor-positive recipients/total number of recipients successfully transplanted) that developed a palpable mass ≥ 5 mm in diameter was compared on day 62 after injection of wild type (WT) or knockout (KO) cells. The asterisks indicate a statistically significant difference between genotypes per cell density determined by Fisher's exact test. Figure S10 Mean WT and KO tumor volume in the 200- and 500-cell input cohorts up to day 36 posttransplant . Comparison of mean tumor volume ± SEM when 500 or 200 wild type (WT) or knockout (KO) cells were injected into a single, cleared inguinal mammary fat pad per recipient. All recipients in the 500- and 200-cell input cohorts were euthanized on day 36 posttransplant to obtain tissue sections and RNA during the early stages of tumor outgrowth. The number of recipients with palpable masses per genotype per density is reviewed in Table 1. Figure S11 Mean tumor volume in the 100-cell WT and KO cohorts up to day 112 posttransplant . Comparison of wild-type (WT) and knockout (KO) mean tumor volume ± SEM over time when 100 cells were injected into a single, cleared inguinal mammary fat pad. The decrease in mean tumor volume observed at day 112 for the 100-cell KO group is due to the exclusion of a subset of individual recipients in this cohort that developed tumors > 500 mm 3 . These recipients were subjected to surgical resection of the primary tumor between days 96 and 112 posttransplant. Figure S12 Mean WT and KO tumor volume in the 10-, 25- and 50-cell input cohorts up to day 112 posttransplant . Comparison of wild type (WT) and knockout (KO) mean tumor volume ± SEM over time when 10, 25 or 50 WT or KO cells were injected into a single, cleared inguinal mammary fat pad. The decrease in mean tumor volume observed at day 112 for the 100-cell KO group is due to the exclusion of a subset of individual recipients in this cohort that developed tumors > 500 mm 3 . These recipients were subjected to surgical resection of the primary tumor between days 96 and 112 posttransplant.
GUID: BD6E9581-BD53-4997-B8E4-1F2C10B39AC3
Rockwell S, Dobrucki IT, Kim EY, Marrison ST, Vu VT. Hypoxia and radiation therapy: past history, ongoing research, and future promise. Curr Mol Med. 2009; 9 :442–458. doi: 10.2174/156652409788167087. [ PMC free article ] [ PubMed ] [ CrossRef ] [ Google Scholar ] Cosse JP, Michiels C. Tumour hypoxia affects the responsiveness of cancer cells to chemotherapy and promotes cancer progression. Anticancer Agents Med Chem. 2008; 8 :790–797. [ PubMed ] [ Google Scholar ] Vaupel P, Höckel M, Mayer A. Detection and characterization of tumor hypoxia using pO 2 histography. Antioxid Redox Signal. 2007; 9 :1221–1235. doi: 10.1089/ars.2007.1628. [ PubMed ] [ CrossRef ] [ Google Scholar ] Semenza GL. Defining the role of hypoxia-inducible factor 1 in cancer biology and therapeutics. Oncogene. 2010; 29 :625–634. doi: 10.1038/onc.2009.441. [ PMC free article ] [ PubMed ] [ CrossRef ] [ Google Scholar ] Zhong H, De Marzo AM, Laughner E, Lim M, Hilton DA, Zagzag D, Buechler P, Issacs WB, Semenza GL, Simons JW. Overexpression of hypoxia-inducible factor 1α in common human cancers and their metastases. Cancer Res. 1999; 59 :5830–5835. [ PubMed ] [ Google Scholar ] Bos R, Zhong H, Hanrahan CF, Mommers E, Semenza GL, Pinedo HM, Abeloff MD, Simons JW, van Diest PJ, van der Wall E. Levels of hypoxia-inducible factor-1α during breast carcinogenesis. J Natl Cancer Inst. 2001; 93 :309–314. doi: 10.1093/jnci/93.4.309. [ PubMed ] [ CrossRef ] [ Google Scholar ] Gort EH, Groot AJ, van der Wall E, van Diest PJ, Vooijs MA. Hypoxic regulation of metastasis via hypoxia-inducible factors. Curr Mol Med. 2008; 8 :60–67. doi: 10.2174/156652408783565568. [ PubMed ] [ CrossRef ] [ Google Scholar ] Chi JT, Wang Z, Nuyten DS, Rodriguez EH, Schaner ME, Salim A, Wang Y, Kristensen GB, Helland A, Børresen-Dale AL, Giaccia A, Longaker MT, Hastie T, Yang GP, van de Vijver MJ, Brown PO. Gene expression programs in response to hypoxia: cell type specificity and prognostic significance in human cancers. PLoS Med. 2006; 3 :e47. doi: 10.1371/journal.pmed.0030047. [ PMC free article ] [ PubMed ] [ CrossRef ] [ Google Scholar ] Dales JP, Garcia S, Meunier-Carpentier S, Andrac-Meyer L, Haddad O, Lavaut MN, Allasia C, Bonnier P, Charpin C. Overexpression of hypoxia-inducible factor HIF-1α predicts early relapse in breast cancer: retrospective study in a series of 745 patients. Int J Cancer. 2005; 116 :734–739. doi: 10.1002/ijc.20984. [ PubMed ] [ CrossRef ] [ Google Scholar ] Atkinson RL, Zhang M, Diagaradjane P, Peddibhotla S, Contreras A, Hilsenbeck SG, Woodward WA, Krishnan S, Chang JC, Rosen JM. Thermal enhancement with optically activated gold nanoshells sensitizes breast cancer stem cells to radiation therapy. Sci Transl Med. 2010; 2 :55ra79. doi: 10.1126/scitranslmed.3001447. [ PMC free article ] [ PubMed ] [ CrossRef ] [ Google Scholar ] Lacerda L, Pusztai L, Woodward WA. The role of tumor initiating cells in drug resistance of breast cancer: implications for future therapeutic approaches. Drug Resist Updat. 2010; 13 :99–108. doi: 10.1016/j.drup.2010.08.001. [ PubMed ] [ CrossRef ] [ Google Scholar ] Creighton CJ, Li X, Landis M, Dixon JM, Neumeister VM, Sjolund A, Rimm DL, Wong H, Rodriguez A, Herschkowitz JI, Fan C, Zhang X, He X, Pavlick A, Gutierrez MC, Renshaw L, Larionov AA, Faratian D, Hilsenbeck SG, Perou CM, Lewis MT, Rosen JM, Chang JC. Residual breast cancers after conventional therapy display mesenchymal as well as tumor-initiating features. Proc Natl Acad Sci USA. 2009; 106 :13820–13825. doi: 10.1073/pnas.0905718106. [ PMC free article ] [ PubMed ] [ CrossRef ] [ Google Scholar ] Mohyeldin A, Garzón-Muvdi T, Quiñones-Hinojosa A. Oxygen in stem cell biology: a critical component of the stem cell niche. Cell Stem Cell. 2010; 7 :150–161. doi: 10.1016/j.stem.2010.07.007. [ PubMed ] [ CrossRef ] [ Google Scholar ] Takubo K, Goda N, Yamada W, Iriuchishima H, Ikeda E, Kubota Y, Shima H, Johnson RS, Hirao A, Suematsu M, Suda T. Regulation of the HIF-1α level is essential for hematopoietic stem cells. Cell Stem Cell. 2010; 7 :391–402. doi: 10.1016/j.stem.2010.06.020. [ PubMed ] [ CrossRef ] [ Google Scholar ] Li Z, Bao S, Wu Q, Wang H, Eyler C, Sathornsumetee S, Shi Q, Cao Y, Lathia J, McLendon RE, Hjelmeland AB, Rich JN. Hypoxia-inducible factors regulate tumorigenic capacity of glioma stem cells. Cancer Cell. 2009; 15 :501–513. doi: 10.1016/j.ccr.2009.03.018. [ PMC free article ] [ PubMed ] [ CrossRef ] [ Google Scholar ] Wang Y, Liu Y, Malek SN, Zheng P. Targeting HIF1α eliminates cancer stem cells in hematological malignancies. Cell Stem Cell. 2011; 8 :399–411. doi: 10.1016/j.stem.2011.02.006. [ PMC free article ] [ PubMed ] [ CrossRef ] [ Google Scholar ] Matsumoto K, Arao T, Tanaka K, Kaneda H, Kudo K, Fujita Y, Tamura D, Aomatsu K, Tamura T, Yamada Y, Saijo N, Nishio K. mTOR signal and hypoxia-inducible factor-1α regulate CD133 expression in cancer cells. Cancer Res. 2009; 69 :7160–7164. doi: 10.1158/0008-5472.CAN-09-1289. [ PubMed ] [ CrossRef ] [ Google Scholar ] Soeda A, Park M, Lee D, Mintz A, Androutsellis-Theotokis A, McKay RD, Engh J, Iwama T, Kunisada T, Kassam AB, Pollack IF, Park DM. Hypoxia promotes expansion of the CD133-positive glioma stem cells through activation of HIF-1α oxygen regulation of brain tumor cells. Oncogene. 2009; 28 :3949–3959. doi: 10.1038/onc.2009.252. [ PubMed ] [ CrossRef ] [ Google Scholar ] Kouros-Mehr H, Bechis SK, Slorach EM, Littlepage LE, Egeblad M, Ewald AJ, Pai SY, Ho IC, Werb Z. GATA-3 links tumor differentiation and dissemination in a luminal breast cancer model. Cancer Cell. 2008; 13 :141–152. doi: 10.1016/j.ccr.2008.01.011. [ PMC free article ] [ PubMed ] [ CrossRef ] [ Google Scholar ] Vaillant F, Asselin-Labat ML, Shackleton M, Forrest NC, Lindeman GJ, Visvader JE. The mammary progenitor marker CD61/β3 integrin identifies cancer stem cells in mouse models of mammary tumorigenesis. Cancer Res. 2008; 68 :7711–7717. doi: 10.1158/0008-5472.CAN-08-1949. [ PubMed ] [ CrossRef ] [ Google Scholar ] Wri
Хардкорные фото секса с подружкой
Пожилая Ирина в сарае и на природе
Рыжая пожилая толстуха засунула в пизду кое что