Галерея 2963902
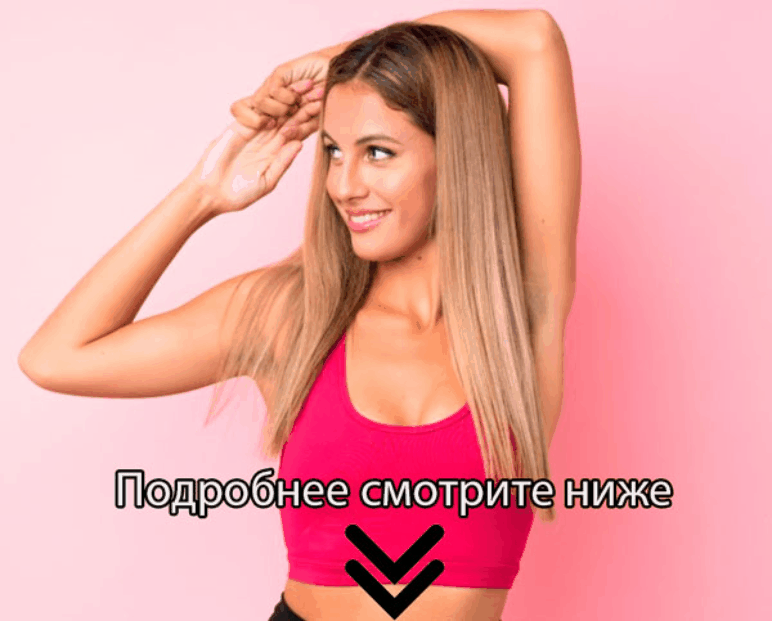
Галерея 2963902
All Books Conferences Courses Journals & Magazines Standards Authors Citations
Abstract: Vertical GaN-on-GaN Schottky barrier diodes based on as-grown and regrown samples were fabricated to investigate the effects of the etch-then-regrow process on device per... View more
Vertical GaN-on-GaN Schottky barrier diodes based on as-grown and regrown samples were fabricated to investigate the effects of the etch-then-regrow process on device performance. The surface roughness increased slightly after dry etching and decreased after regrowth. According to X-ray diffraction results, the etch-then-regrow process caused a slight increase of defect density due to increased edge dislocations. Schottky parameters extracted from forward current-voltage curves, such as turn-on voltages of 0.74 V and 0.72 V, ideality factors of 1.07 and 1.10, and barrier heights of 1.07 eV and 1.05 eV, were obtained for diodes based on the regrown and as-grown samples, respectively. The breakdown voltage of the regrown sample was much lower than the as-grown sample. The regrowth interface can be regarded as a n-doping GaN layer due to the high interface charge density after the etch-then-regrown process. This equivalent
{n}
-doping GaN layer reduced the effective thickness of the UID-GaN under the Schottky contact thus causing lower breakdown voltage for the regrown sample. Poole-Frenkel emission and trap-assisted tunneling processes were responsible for the leakage of both as-grown and regrown samples according to the temperature dependence of the reverse currents.
Date of Publication: 03 January 2020
TABLE 1
Leakage Processes and the Expressions
T. Kachi, "Recent progress of GaN power devices for automotive applications", Jpn. J. Appl. Phys. , vol. 53, Sep. 2014.
K. J. Chen et al., "GaN-on-Si power technology: Devices and applications", IEEE Trans. Electron. Devices , vol. 64, pp. 779-795, Mar. 2017.
I. C. Kizilyalli, P. Bui-Quang, D. Disney, H. Bhatia and O. Aktas, "Reliability studies of vertical GaN devices based on bulk GaN substrates", Microelectron. Rel. , vol. 55, no. 9, pp. 1654-1661, Aug./Sep. 2015.
I. C. Kizilyalli, T. Prunty and O. Aktas, "
4-kV and 2.8-
text{m}Omega
-cm
2
vertical GaN
p-n
diodes with low leakage currents
", IEEE Electron Device Lett. , vol. 36, no. 10, pp. 1073-1075, Oct. 2015.
M. Sun, Y. Zhang, X. Gao and T. Palacios, "High-performance GaN vertical fin power transistors on bulk GaN substrates", IEEE Electron Device Lett. , vol. 38, no. 4, pp. 509-512, Apr. 2017.
H. Fu et al., "
High performance vertical GaN-on-GaN
p-n
power diodes with hydrogen-plasma-based edge termination
", IEEE Electron Device Lett. , vol. 39, no. 7, pp. 1018-1021, Jul. 2018.
H. Q. Fu, X. Q. Huang, H. Chen, Z. J. Lu, I. Baranowski and Y. J. Zhao, "Ultra-low turn-on voltage and on-resistance vertical GaN-on-GaN Schottky power diodes with high mobility double drift layers", Appl. Phys. Lett. , vol. 111, no. 15, Oct. 2017.
S. O. Kucheyev, J. S. Williams and S. J. Pearton, "Ion implantation into GaN", Mater. Sci. Eng. R Rep. , vol. 33, no. 2, pp. 51-108, May 2001.
Y. Zhang et al., "Vertical GaN junction barrier Schottky rectifiers by selective ion implantation", IEEE Electron Device Lett. , vol. 38, no. 8, pp. 1097-1100, Aug. 2017.
J. K. Sheu and G. C. Chi, "The doping process and dopant characteristics of GaN", J. Phys. Condensed Matter , vol. 14, pp. R657-R702, May 2002.
U. Singisetti et al., "Enhancement-mode N-polar GaN MISFETs with self-aligned source/drain regrowth", IEEE Electron Device Lett. , vol. 32, no. 2, pp. 137-139, Feb. 2011.
J. Guo et al., "
MBE-regrown Ohmics in InAlN HEMTs with a regrowth interface resistance of
0.05~Omegacdot
mm
", IEEE Electron Device Lett. , vol. 33, no. 4, pp. 525-527, Apr. 2012.
Y. Yue et al., "
InAlN/AlN/GaN HEMTs with regrown ohmic contacts and
f_{T}
of 370 GHz
", IEEE Electron Device Lett. , vol. 33, no. 7, pp. 988-990, Jul. 2012.
S. Chowdhury, M. H. Wong, B. L. Swenson and U. K. Mishra, "CAVET on bulk GaN substrates achieved with MBE-regrown AlGaN/GaN layers to suppress dispersion", IEEE Electron Device Lett. , vol. 33, no. 1, pp. 41-43, Jan. 2012.
H. Xing, S. P. DenBaars and U. K. Mishra, "Characterization of AlGaN/GaNp-n diodes with selectively regrown n-AlGaN by metal-organic chemical-vapor deposition and its application to GaN-based bipolar transistors", J. Appl. Phys. , vol. 97, no. 11, 2005.
G. Koblmüller, R. M. Chu, A. Raman, U. K. Mishra and J. S. Speck, "High-temperature molecular beam epitaxial growth of AlGaN/GaN on GaN templates with reduced interface impurity levels", J. Appl. Phys. , vol. 107, no. 4, 2010.
K. Fu et al., "
Investigation of GaN-on-GaN vertical
p-n
diode with regrown p-GaN by metalorganic chemical vapor deposition
", Appl. Phys. Lett. , vol. 113, no. 23, Dec. 2018.
I. Stricklin et al., "
Investigation of interfacial impurities in m-plane GaN regrown
p-n
junctions for high-power vertical electronic devices
", Proc. SPIE , Sep. 2018.
M. Azize, Z. Bougrioua and P. Gibart, "Inhibition of interface pollution in AlGaN/GaN HEMT structures regrown on semi-insulating GaN templates", J. Cryst. Growth , vol. 299, no. 1, pp. 103-108, Feb. 2007, [online] Available: https://doi.org/10.1016/j.jcrysgro.2006.10.250.
W. Lee et al., "Optimization of Fe doping at the regrowth interface of GaN for applications to III-nitride-based heterostructure field-effect transistors", Appl. Phys. Lett. , vol. 90, no. 9, Feb. 2007.
J. P. Liu et al., "III-nitride heterostructure field-effect transistors grown on semi-insulating GaN substrate without regrowth interface charge", Appl. Phys. Lett. , vol. 92, no. 13, Mar. 2008.
Z. Hu et al., "
1.1-kV vertical GaN
p-n
diodes with p-GaN regrown by molecular beam epitaxy
", IEEE Electron Device Lett. , vol. 38, no. 8, pp. 1071-1074, Aug. 2017.
G. W. Pickrell et al., "
Regrown vertical GaN
p-n
diodes with low reverse leakage current
", J. Electron. Mater. , vol. 48, no. 5, pp. 3311-3316, May. 2019.
T. Yamamoto and H. Katayama-Yoshida, "Materials design for the fabrication of low-resistivity p-type GaN using a codoping method", Jpn. J. Appl. Phys. , vol. 36, no. 2, pp. L180-L183, Feb. 1997.
H. Katayama-Yoshida, R. Kato and T. Yamamoto, "New valence control and spin control method in GaN and AlN by codoping and transition atom doping", J. Cryst. Growth , vol. 231, no. 3, pp. 428-436, Oct. 2001.
Y. Akatsuka, S. Iwayama, T. Takeuchi, S. Kamiyama, M. Iwaya and I. Akasaki, "Doping profiles in low resistive GaN tunnel junctions grown by metalorganic vapor phase epitaxy", Appl. Phys. Exp. , vol. 12, no. 2, Feb. 2019.
S. M. Sze and K. K. Ng, Physics of Semiconductor Devices, Hoboken, NJ, USA:Wiley, pp. 170-171, 2007.
A. Kumar, S. Arafin, M. C. Amann and R. Singh, "Temperature dependence of electrical characteristics of Pt/GaN Schottky diode fabricated by UHV e-beam evaporation", Nanoscale Res. Lett. , vol. 8, pp. 481, Nov. 2013.
H. Iwano, S. Zaima and Y. Yasuda, "Hopping conduction and localized states in p-Si wires formed by focused ion beam implantations", J. Vacuum Sci. Technol. B Microelectron. Nanometer Structures Process. Meas. Phenom. , vol. 16, pp. 2551-2554, Jul. 1998.
D. Yu, C. Wang, B. L. Wehrenberg and P. Guyot-Sionnest, "Variable range hopping conduction in semiconductor nanocrystal solids", Phys. Rev. Lett. , vol. 92, May 2004.
P. Mark and W. Helfrich, "Space-charge-limited currents in organic crystals", J. Appl. Phys. , vol. 33, pp. 205-215, Jan. 1962.
J. A. Röhr, D. Moia, S. A. Haque, T. Kirchartz and J. Nelson, "Exploring the validity and limitations of the Mott–Gurney law for charge-carrier mobility determination of semiconducting thin-films", J. Phys. Condens. Matter , vol. 30, Feb. 2018.
M. P. Houng, Y. H. Wang and W. J. Chang, "Current transport mechanism in trapped oxides: A generalized trap-assisted tunneling model", J. Appl. Phys. , vol. 86, no. 3, pp. 1488-1491, Aug. 1999.
Z. H. Liu, G. I. Ng, S. Arulkumaran, Y. K. T. Maung and H. Zhou, "
Temperature-dependent forward gate current transport in atomic-layer-deposited Al
2
O
3
/AlGaN/GaN metal-insulator-semiconductor high electron mobility transistor
", Appl. Phys. Lett. , vol. 98, no. 16, Apr. 2011.
A. S. Barker and M. Ilegems, "Infrared lattice vibrations and free-electron dispersion in GaN", Phys. Rev. B Condens. Matter , vol. 7, pp. 743-750, Jan. 1973.
V. W. L. Chin, T. L. Tansley and T. Osotchan, "Electron mobilities in gallium indium and aluminum nitrides", J. Appl. Phys. , vol. 75, no. 11, pp. 7365-7372, Jun. 1994.
H. Zhang, E. J. Miller and E. T. Yu, "
Analysis of leakage current mechanisms in Schottky contacts to GaN and Al
0.25
Ga
0.75
N/GaN grown by molecular-beam epitaxy
", J. Appl. Phys. , vol. 99, no. 2, Jan. 2006.
C. D. Wang et al., "Deep level defects in n-type GaN grown by molecular beam epitaxy", Appl. Phys. Lett. , vol. 72, no. 10, pp. 1211-1213, Mar. 1998.
IEEE Account
Change Username/Password
Update Address
Purchase Details
Payment Options
Order History
View Purchased Documents
Need Help?
US & Canada: +1 800 678 4333
Worldwide: +1 732 981 0060
Contact & Support
Gallium nitride (GaN) has become one of the most important materials for power electronics, due to its superior physical and electrical properties such as large bandgap, high breakdown electric field, high operation temperature and high electron velocity. These characteristics make GaN-based devices capable of remarkable improvements in energy conversion efficiency, increased switching frequency, and reduced electric system volume [1] , [2] . Furthermore, as GaN substrates are becoming commercially available, vertical devices grown on GaN substrates have emerged as highly promising candidates for high-performance power electronics due to dramatically reduced density of dislocations [3] – [7] .
Meanwhile, in order to achieve the best performance and flexibility in device structure design, selective-area doping is indispensable. Compared with ion implantation [8] , [9] and diffusion [10] , selective epitaxial regrowth is still the most effective ways for GaN devices. A lot of work on regrown {n} ^{+}
-GaN have been reported that the contact resistance could be dramatically reduced [11] – [13] . Regrown AlGaN/GaN layers was reported to suppress the dispersion of GaN vertical transistors [14] . However, one big challenge for the regrowth is the interface contamination, including Si, O, and C [15] – [18] . The C and O contaminants could be effectively reduced by in situ thermal cleaning [16] . But the Si contamination is difficult to remove even after using Fe or C doping for the semi-insulating substrate or buffer layer [16] , [19] – [21] , and the origin is still unclear. Although the semi-insulating substrate or buffer layer is useful for reducing leakage current, no leakage performance above 60 V was found in the reported results [16] , [19] – [21] . The existence of Si at the interface may not be a problem for the regrown {n} ^{+}
-GaN as ohmic contacts because the Si is the very donor used for {n}
-doping. But this is a big problem for the regrowth of {p}
-GaN. Hu et al. investigated the performance of vertical GaN p-n diodes with regrown {p}
-GaN, reaching breakdown voltage of 1.1 kV [22] . However, they did the regrowth on a non-etched surface whereas dry etching is almost inevitable in selective regrowth. Recently, Pickrell et al. found that regrown diodes including dry etching process prior to the {p}
-GaN regrowth showed a large Si spike at the regrowth interface and demonstrated significantly higher reverse leakage current than the as-grown diodes [23] . However, they only showed simple current-voltage curves for the etch-then-regrow diodes. Few reports were found on the details of the effects of the etch-then-regrow process for now. Meanwhile, the co-doping or interaction effect of donors (Si) and acceptors (Mg) could make the interface issue more complex [24] – [26] .
To simplify the question and get a direct understanding of the interface issues after the etch-then-grow process, we did the etch-then-grow process by regrowing unintentionally doped (UID) GaN on UID-GaN in this work without the influences of the co-doping or interaction effect of donors and acceptors. In addition to the influences of the etch-then-regrow process on material properties, vertical GaN-on-GaN Schottky barrier diodes were fabricated on both as-grown and regrown samples to investigate the effect of the regrowth interface on device leakage and breakdown.
Both the as-grown and regrown samples were homoepitaxially grown by metalorganic chemical vapor deposition (MOCVD) on 2-inch {c}
-plane {n} ^{+}
-GaN ( 1\times 10^{18}
cm ^{-3}
) free-standing substrates which were grown by hydride vapor phase epitaxy (HVPE). 2- \mu \text{m}
-thick UID-GaN epilayers were first grown on the GaN substrates for both samples. The UID-GaN for the regrown sample was first etched off 500 nm by the inductively coupled plasma (ICP) etching. Then, another 500-nm-thick UID-GaN was regrown on the etched surface using the same growth condition with the original 2- \mu \text{m}
-thick UID-GaN without any other surface treatment. The etch-then-regrow process is shown in Fig. 1 . Surface roughnesses, measured by atomic force microscopy (AFM), of the as-grown sample, the sample after etching, and the sample after regrowth, are 0.157 nm, 0.207 nm and 0.137 nm, respectively. This means the surface roughness increased slightly after dry etching but was recovered after the regrowth. The increased surface roughness after dry etching also indicates that etching damage could be introduced by the process. Although the etching damage and corresponding surface roughness can be reduced by optimizing the etching condition, the purpose of this work is to investigate the effect of the dry etching damage on the device performance.
Schematics of (a) as-grown sample, (b) sample after etching, and (c) sample after regrowth. The AFM images below compare surface morphology with a scanning area of 5\,\,{\mu }{\mathrm{ m}} \times 5 { \mu }{\mathrm{ m}}
.
Vertical GaN-on-GaN Schottky barrier diodes (SBDs) based on the as-grown and regrown samples were fabricated by an identical process. Ni/Pt/Au (10 nm/30 nm/120 nm) metal stacks were deposited by electron-beam evaporation for Schottky contacts with a diameter of 120~\mu \text{m}
. Mesa isolation, with a diameter of 180~\mu \text{m}
and etch depth of 1.5~\mu \text{m}
, was done by chlorine-based ICP etching. Metal stacks of Ti/Al/Ni/Au (20 nm/130 nm/50 nm/50 nm) were deposited for ohmic contacts on the backside of the GaN substrate. Forward current-voltage ( I-V ) and capacitance-voltage ( C-V ) characteristics were measured using a Keithley 4200-SCS parameter analyzer and reverse I-V characteristics were measured by a Keithley 2410 sourcemeter.
Fig. 2(a) and 2(c) show X-ray diffraction (XRD) omega rocking curves for symmetric (002) and asymmetric (102) reflections, respectively. The full-width-half-maximum (FWHM) for the (002) and (102) reflections are summarized in Figs. 2(b) and 2(d) , respectively. The FWHM of the (002) reflection for the regrown sample is almost the same as the as-grown sample. On the other hand, an increase of the FWHM for the (102) reflection after the etch-then-regrow process is obvious ( Fig. 2(d) ). The results indicate that the etch-then-regrow process mostly influenced edge dislocations because the FWHM for (002) and (102) reflections are typically related to screw and edge threading dislocations, respectively. Similar results on the increase of the FWHM for the (102) reflection were also found in the regrown {p}
-GaN after the etch-then-regrow process [17] . Dislocation densities for the as-grown sample, the sample after etching, and the sample after regrowth are shown in Fig. 3(a) . The dislocation densities are all in the range of 10^{6}
cm ^{-3}
with a slight increase after the etch-then-regrow process. However, no clear regrowth interface was visible by transmission electron microscopy (TEM) in this work ( Fig. 3(b) ). This is quite different from the regrown {p}
-GaN after the etch-then-regrow process where an interfacial layer at the regrowth interface was clearly observed [17] . Further work is being done to find the reason.
(a) XRD omega rocking curves of (002) reflection of as-grown (red line), etched (pink dash) and regrown samples (blue dash dot), and (b) corresponding FWHM. (c) XRD omega rocking curves of (102) reflections, and (d) corresponding FWHM.
(a) Dislocation densities according to XRD. (b) TEM image of the regrown sample.
Fig. 4(a) shows the forward I-V characteristics for both samples. Turn-on voltages for the as-grown and regrown samples, extracted by linear extrapolation, are 0.74 V and 0.72 V, respectively.
(a) Current density in linear scale, and on-resistance in logarithmic scale for as-grown (red), and regrown (blue) samples, as a function of forward bias. Short dash lines indicate turn-on voltages by linear extrapolation. (b) Current density vs. forward bias with semi-log scale. Ideality factor and saturation current density were extracted from linear segments.
The reverse I–V characteristics of the samples are shown in Fig. 5 . The breakdown voltages of the as-grown and regrown samples were 282 V and 89 V, respectively. The breakdown was not due to avalanche for both samples since the breakdown voltage did not increase with the temperature. To investigate the influences of the regrowth interface on the breakdown voltage, charge density profiles of the as-grown and regrown samples were extracted from C-V curves at different frequencies ( Fig. 6 ). The charge density of the UID-GaN for the as-grown sample is in the range of 10^{14} - 10^{16}
cm ^{-3}
. For the regrown sample, the charge density shows a spike up to 10^{19}
cm ^{-}{}^{3}
at the depth of ~ 500 nm, where is exactly the location of the regrowth interface. The high charge density is believed to be attributed to the donor-like contaminants, such as Si and O [15] – [18] .
Reverse I-V curves of Schottky barrier diodes on as-grown (red line), and regrown (blue line) samples.
Charge density profiles of (a) as-grown sample, and (b) regrown sample extracted from C-V results at different frequencies.
To further reveal the effects of the regrowth interface on the device performance, we did device simulation using Silvaco. Based on the discussion above, the regrowth interface was treated as a {n}
-doping GaN layer in the simulation. The thickness of the interfacial layer is set to 100 nm, which is estimated from the charge density profile and secondary-ion mass spectrometry (SIMS). However, the thickness of the interfacial layer is not so importa
Анальный секс с плоской брюнеткой
Блондинка дала полизать парню пальцы ног
Пуэрториканский транс Алана Лопез