Галерея 2763124
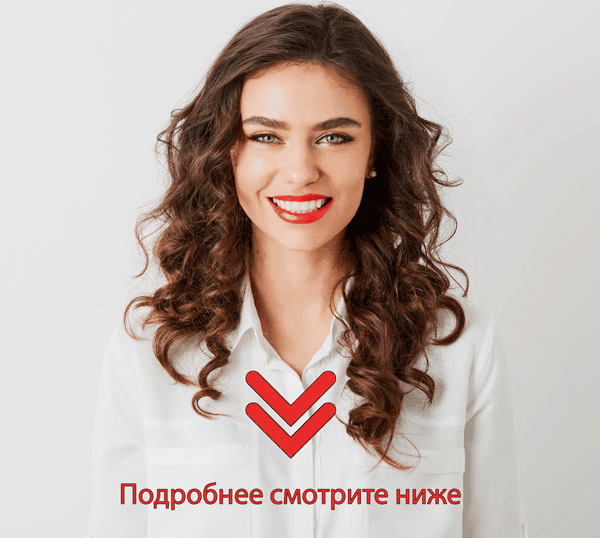
🛑 ПОДРОБНЕЕ ЖМИТЕ ЗДЕСЬ 👈🏻👈🏻👈🏻
Галерея 2763124
Issues
Topics
For Authors
About
Editorial Board
Journals Home
Jump To...
Methods
Results
Discussion
Acknowledgments
References
Protection of the Retinal Ganglion Cells: Intravitreal Injection of Resveratrol in Mouse Model of Ocular Hypertension
Department of Ophthalmology and Visual Science, Tokyo Medical and Dental University, Tokyo, Japan
Department of Ophthalmology and Visual Science, Tokyo Medical and Dental University, Tokyo, Japan
Department of Ophthalmology and Visual Science, Tokyo Medical and Dental University, Tokyo, Japan
Department of Ophthalmology and Visual Science, Tokyo Medical and Dental University, Tokyo, Japan
Department of Ophthalmology and Visual Science, Tokyo Medical and Dental University, Tokyo, Japan
Department of Ophthalmology and Visual Science, Tokyo Medical and Dental University, Tokyo, Japan
Department of Ophthalmology and Visual Science, Tokyo Medical and Dental University, Tokyo, Japan
Department of Ophthalmology and Visual Science, Tokyo Medical and Dental University, Tokyo, Japan
Correspondence: Takeshi Yoshida, Department of Ophthalmology and Visual Science, Tokyo Medical and Dental University, 1-5-45 Yushima Bunkyo-ku, Tokyo 1138519, Japan; takec.oph@tmd.ac.jp .
Investigative Ophthalmology & Visual Science March 2020, Vol.61, 13. doi: https://doi.org/10.1167/iovs.61.3.13
Protection of the Retinal Ganglion Cells: Intravitreal Injection of Resveratrol in Mouse Model of Ocular Hypertension
You will receive an email whenever this article is corrected, updated, or cited in the literature. You can manage this and all other alerts in My Account
This feature is available to authenticated users only.
Sign In
or
Create an Account
×
Glaucoma is the leading cause of irreversible blindness worldwide. 1 , 2 The disease is characterized by progressive degeneration of retinal ganglion cells (RGCs) projecting into the brain. 3 , 4 A major risk factor for this disease is elevated intraocular pressure (IOP). 5 Currently, the only clinically effective glaucoma treatment is oriented toward lowering IOP. 6 , 7 Neuroprotective interventions against glaucoma, which may have therapeutic potential, have not been thoroughly explored. 8 , 9
Oxidative stress (OS) generates reactive oxygen species (ROS). 10 – 12 Recent studies using experimental glaucoma models and clinical samples have demonstrated that OS is present in ocular tissue, indicating its potential role in the pathogenesis of glaucoma. 13 , 14 The mechanisms by which OS may induce RGC loss are not fully understood but may include direct neurotoxic effects from ROS or indirect damage from OS-induced dysfunction of glial cells. 15 Tumor suppressor p53 is a redox active transcription factor that organizes and directs cellular responses against a variety of stresses; it regulates the cell cycle, in particular by eliminating cells with lethal insults via induction of apoptosis. 16 Guo et al. 17 demonstrated that an increase in retinal p53 mRNA levels is positively correlated with optic nerve injury due to elevated IOP. Experimentally, it has been shown that inhibitors and enhancers of p53 modulate RGCs survival. 18 , 19 Furthermore, clinical studies have revealed associations between specific p53 haplotypes and polymorphisms with the progression of primary open-angle glaucoma in human, 20 – 22 emphasizing the potential importance of p53 in RGCs death. Recent studies have revealed that cell-generated ROS and p53 are implicated in mediating apoptosis. 23 , 24
Resveratrol (RSV), a natural polyphenolic compound, is known as an activator of Sirtuin 1 (Sirt1), a class III histone deacetylase dependent on nicotinamide adenine dinucleotide. Sirt1 regulates cell senescence, DNA damage repair, and apoptosis and controls longevity in response to caloric restriction in numerous mammals. 25 – 27 It is therefore thought to have several health-enhancing properties. Indeed, many clinical studies have highlighted the beneficial effects of RSV in human diseases. 28 – 32 RSV has also been suggested as a protective agent in preventing and treating organ injury due to its antioxidative properties. 33 – 36 Pirhan et al. 37 showed that intraperitoneal administration of RSV delays RGC loss in an experimental glaucoma model; however, the underlying molecular mechanisms behind this phenomenon are unknown. Notable progress has been made in identifying the critical role of retinal Müller glial cells (MGCs) in maintaining retinal homeostasis through the expression of various neuroprotective signaling molecules 38 – 40 protecting the retina from many stresses, including OS. 41 Among various neuroprotective signaling molecules that MGCs express, brain-derived neurotrophic factor (BDNF) is known to be a potent neurotrophic molecule. Harada et al. 42 , 43 previously reported that brimonidine, a selective α 2 -adrenergic receptor agonist, stimulates the expression of BDNF in MGCs and promotes RGC survival. In the present study, we used a murine model of high IOP to characterize and study the implications of retinal OS and p53 expression on IOP-induced RGC cell death in response to intravitreal RSV administration. Using this model, we also studied the changes in the expression of BDNF in MGCs in response to intravitreal administration of RSV. We revealed that intravitreal injection of RSV upregulates Sirt1 expression in RGCs and rescues RGCs from high IOP-induced RGCs cell death by multiple pathways, including reduced ROS generation and acetyl-p53 expression in RGCs. We also revealed that intravitreal injection of RSV activates MGCs and upregulates a potent neurotrophic factor, BDNF, to rescue RGCs from cell death induced by high IOP.
All animal study protocols were approved by the Animal Care and Use Committee of Tokyo Medical and Dental University (Permit No. A2017-328A). All procedures were carried out in accordance with the Association for Research in Vision and Ophthalmology Statement for the Use of Animals in Ophthalmic and Vision Research. A total of 75 adult male C57BL/6JJcl mice (CLEA Japan, Tokyo, Japan) were divided into five groups: control, microbead injection, microbead injection with 3-µM RSV, microbead injection with 30-µM RSV, and 30-µM RSV injection ( n = 15 in each group). All mice were anesthetized using 40 mg/kg pentobarbital (Kyouritu Seiyaku Corporation, Tokyo, Japan), and their pupils were dilated with 0.5% phenylephrine hydrochloride and 0.5% tropicamide (Santen Pharmaceutical Co., Ltd., Tokyo, Japan).
Induction and Measurement of IOP Model
High IOP was induced using microbeads as described in previous studies. 3 , 44 , 45 Microbead-injected mice groups received 3 µl of 10-µm-diameter polystyrene microbeads (Polysciences, Inc., Warrington, PA, USA) at a final concentration of 4.55 × 10 6 microbeads per milliliter, via a 35-gauge needle, into the anterior chamber of the right eye. Mice in the control group received an injection of the same volume, but of Gibco PBS (Thermo Fisher Scientific, Waltham, MA, USA). IOP was measured every second day using a Tono-Pen AVIA tonometer (Reichert Technologies, Depew, NY, USA). Prior to measurement, mice were anesthetized using pentobarbital. The mean of six readings in each eye was utilized to calculate IOP.
Mice were administered 1 µl of 3-µM or 30-µM RSV (Tokyo Chemical Industry Co., Ltd., Tokyo, Japan) dissolved in PBS by injection into the right eye with a 35-gauge needle in the vitreous body on the same day after the microbead injection. As control, 1 µl of PBS was injected into the left eye. To prevent infection, ofloxacin ointment (Santen Pharmaceutical Co., Osaka, Japan) was applied.
Flat-Mount Retina Immunohistochemistry
To make the retinal flat-mounts, mice eyes were enucleated and fixed in 4% paraformaldehyde overnight at 4°C. The cornea and lens were removed, and the entire retina was carefully dissected from the eyecup. Radial cuts (average of four) were made from the edge of the eyecup to the equator and then washed with PBS containing 0.5% Triton X-100 (Bio-Rad, California, USA), as described previously. 46 – 48 For Brn-3a-positive RGC counts on flat-mounts, the retinas were stained overnight at 4°C with mouse anti-Brn-3a monoclonal antibody (1:100; Santa Cruz Biotechnology, Dallas, TX, USA). The retinas were then incubated with anti-mouse IgG for 2 hours at room temperature (RT). For quantification of Brn-3a-positive RGCs, each retinal quadrant was further subdivided into regions of acquisition defined as the peripheral retina (the retinal portion 2.5 mm from the optic nerve head) or the central retina (the retinal portion in the nearby area, 1 mm from the optic nerve head). We took eight images (290 µm × 290 µm) each of the peripheral retina and central retina (total four images per quadrant, 16 images per eye) under masked conditions using a confocal microscope (SP8; Leica Camera AG, Wetzlar, Germany). In this way, labeled cells were identified, counted, and pooled together, as described previously. 49 – 52 All data were quantified using ImageJ software v1.51 (National Institutes of Health, Bethesda, MD, USA) as previously described, 53 – 55 which automatically calculated the number of Brn-3a-positive cells per image and the density of Brn-3a cells/mm 2 of retina in the five groups.
All mice were euthanized at 21 days after microbead and/or RSV injection, as previously reported. 45 , 56 – 58 To prepare frozen sections, eyes were enucleated and embedded in optimal cutting temperature (OCT) compound (Sakura Finetek Japan, Tokyo, Japan) frozen in liquid nitrogen. The frozen blocks were cut into 10-µm sagittal retinal sections with a full length of retina through the ora serrata and optic nerve, using a Leica CM3050 S Cryostat, as previously described. 59 , 60 To make paraffin sections, the eyes were enucleated and dipped in Carnoy's solution (absolute ethanol:chloroform:glacial acetic acid, 6:3:1) for 3 hours at 4°C and then dehydrated and embedded in paraffin for processing to 4-µm paraffin sections. 59 , 60 We selected the sections with a full length of retina through the ora serrata and optic nerve and observed regions at a distance of 100 to 200 µm from the center of the optic nerve in all radial retinal sections, as described previously. 61 Four regions from six sections per retina were prepared and used for statistical analysis.
To detect the generation of ROS in retina, fresh frozen sections incubated with 0.1 µmol/L dihydroethidium (Invitrogen Molecular Probes, Thermo Fisher Scientific) in a light-protected, humidified chamber at 37°C for 30 minutes, as described previously. 62 Nuclei were stained with 4′,6-diamidino-2-phenylindole (DAPI; Vector Laboratories, Inc., Burlingame, CA, USA).
For the detection of apoptotic RGCs, terminal deoxynucleotidyl transferase dUTP nick end labeling (TUNEL) staining was performed using an in situ cell death detection kit (Roche, Mannheim, Germany), as described previously. 63 Before staining, the paraffin sections were dewaxed, rehydrated, and incubated with a proteinase K working solution (Wako Pure Chemical Industries, Ltd., Osaka Japan) per the manufacturer's protocol. Fluorescein isothiocyanate immunofluorescence was then used, and the nuclei were co-stained with DAPI.
Paraffin sections were dewaxed, rehydrated, and heated in a plastic jar containing 200 ml of 0.1-M citrate buffer with a pH of 6.0 under microwave irradiation for 10 minutes at 350 W. Sections were cooled to RT and washed three times with PBS. The sections were blocked in 10% normal goat serum (Vector Laboratories) in PBS and incubated with mouse anti-Brn-3a monoclonal antibody (1:100; Santa Cruz Biotechnology), rabbit anti-Sirt1 primary antibody (1:200; Cell Signaling Technology, Inc., Boston, MA, USA), mouse anti-neurofilament H (clone SMI32) primary antibody (1:1000; Covance, Princeton, NJ, USA), rabbit anti-glia fibrillary acidic protein (GFAP) antibody (1:1; Dako, Carpentaria, CA, USA), mouse anti-BDNF antibody (1:100; Abcam, Cambridge, MA, USA), anti-glutamine synthetase antibody (1:5000; Abcam), and rabbit anti-tropomyosin receptor kinase B (TrkB) antibody (1:250; Abcam) overnight at 4°C. Anti-acetyl-p53 (1:200; Flarebio Biotech LLC, College Park, MD, USA) was stained using a fluorescein-based M.O.M. Immunodetection Kit (Vector Laboratories). The sections were washed with PBS three times before they were incubated with an appropriate fluorophore-conjugated secondary antibody for 1 hour. All sections were mounted with DAPI and imaged with an SP8 confocal microscope, and all data were quantified using ImageJ software, as described earlier. 64 , 65
MGCs were isolated from murine pup eyes (postnatal days 4–5) and cultured as described previously. 42 In brief, MGCs were seeded onto 35-mm dishes (Ibidi GmbH, Martinsried, Germany) and cultured in Dulbecco's Modified Eagle's Medium (DMEM; Sigma-Aldrich, St. Louis, MO, USA) containing 100 U/ml penicillin, 100 µg/ml streptomycin (Wako Pure Chemical Industries), and 10% fetal bovine serum (FBS; Biowest, Nuaille, France). Cultures were maintained at 37°C in a humidified 95% air/5% CO 2 atmosphere.
The viability of MGCs was determined using a Cell Counting Kit-8 (CCK-8) assay (Dojindo Laboratories, Kumamoto, Japan), as in previous studies. 66 , 67 The isolated and cultured mouse MGCs were plated in 96-well plates (5 × 10 3 cells per well) and incubated for 24 hours. RSV was diluted in DMEM with 0.2% dimethyl sulfoxide (Sigma-Aldrich), and the vehicle was used as control. MGCs were then treated with different concentrations of RSV (3, 30, 70, and 140 µM) in culture medium for 6 hours. Then, 10 µl of CCK-8 solution was added to each well in the dark followed by incubation for 2 hours at 37°C. Measurements of optical density were obtained at a wavelength of 450 nm using a microplate reader (Bio-Rad Laboratories, Inc., Hercules, CA, USA). Each experiment was performed in triplicate.
One-hundred percent confluent MGCs were incubated with 3-, 30-, 70-, or 140-µM RSV for 6 hours following the previous study protocol, 40 as well as cell viability assay. Total RNA was extracted from cultured MGCs using an illustra RNAspin Mini RNA Isolation Kit (GE Healthcare, Amersham, UK). cDNA was synthesized from 1 µg total RNA using the ReverTra Ace (Toyobo Co., Osaka, Japan) according to the manufacturer's instructions. Semiquantitative PCR was performed using KAPA SYBR FAST qPCR Master Mix (Kapa Biosystems, Inc., Boston, MA, USA), and a Roche LightCycler 480 Instrument II system was used to detect the gene expression of BDNF. We used a β-actin primer as a normalization factor that was commercially purchased (Qiagen, Hilden, Germany). The amplification schedule was as follows: initial denaturation at 95°C, followed by 45 cycles of 95°C for 30 seconds, 55°C (BDNF and β-actin) for 1 minute, and 72°C for 30 seconds. The following primer sequences were used: BDNF forward, 5′-ctgagcgtgtgtgacagtatt-3′, and reverse, 5′-ctttggataccgggactttctc-3′ (GenBank accession number: NM_001316310.1). The relative change in mRNA expression was calculated using ΔΔCT values, and each experiment was performed in triplicate. Levels were normalized to those of β-actin and reported as fold change compared with the controls.
The protein expression of Sirt1, acetyl-p53, BDNF, and TrkB in the whole retina were analyzed by western blot. After mice eyeballs were enucleated, the retina and sclera were isolated. Protein lysates were extracted using RIPA Lysis Buffer (Thermo Fisher Scientific) supplemented with protease inhibitor (Sigma-Aldrich), according to the manufacturers’ protocol. Briefly, all lysates were quantified, and equal volumes were loaded on NuPAGE 4–12% Bis-Tris gel (Invitrogen) before being transferred to polyvinylidene difluoride membranes (Invitrogen). Membranes were blocked with 3% skim milk and incubated with anti-Sirt1 primary antibodies (1:1000; Cell Signaling Technology), anti-acetyl-p53 (1:400; Abcam), rabbit anti-BDNF antibody (1:500; GeneTex, San Antonio, TX, USA), and rabbit anti-TrkB antibody (1:1000; Abcam) overnight at 4°C. Bound proteins were detected using peroxidase-conjugated ECL anti-mouse IgG and anti-rabbit (GE Healthcare UK). Proteins were visualized using a chemiluminescence substrate (Bio-Rad Laboratories) and imaging system (DNR Bio Imaging Systems, Neve Yamin, Israel). Glyceraldehyde-3-phosphate dehydrogenase was used as a loading control. The expression ratios of protein bands were quantified using ImageJ, as previous described. 68
Quantitative data were expressed as mean ± SD. Data analysis was performed by SPSS Statistics 24.0 (IBM, Armonk, NY, USA) using Student's t -test, one-way ANOVA, and Tukey post hoc test to compare two or more groups. Statistical differences were considered significant for P < 0.05.
Change in Intraocular Pressure After Microbead Injection
The IOP of the mice was measured every 2 days following the microbead injections. Mice in the control group that received an injection of PBS exhibited a steady IOP level (9.8 ± 1.5 mm Hg) throughout the experimental period. Compared with the control group, an elevation in IOP was induced at 4 days after injection in the microbead and
Анал в колготках и чулках
Не понятно кто тут главный
Эшли и Маббель отдыхают на природе вместе