Charge Coupled
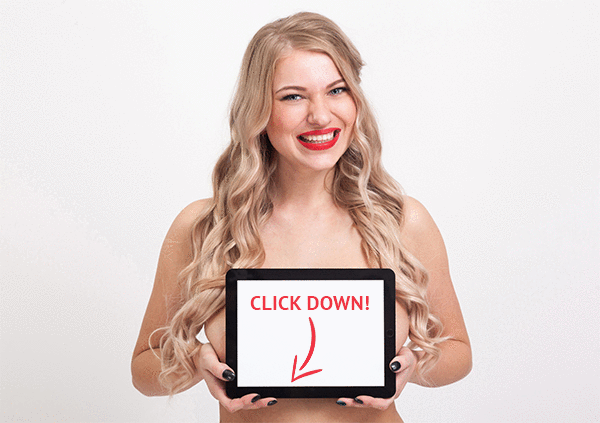
⚡ ALL INFORMATION CLICK HERE 👈🏻👈🏻👈🏻
Charge Coupled
Answers
Definitions
Essential Guides
Opinions
Photo Stories
Podcasts
Quizzes
Tech Accelerators
Tutorials
Sponsored Communities
Home
Architecture & Strategy
Healthcare IT
charge-coupled device
This was last updated in November 2021
Related Terms
binary
Binary describes a numbering scheme in which there are only two possible values for each digit -- 0 or 1 -- and is the basis for ...
See complete definition
data lifecycle management (DLM)
Data lifecycle management (DLM) is a policy-based approach to managing the flow of an information system's data throughout its ...
See complete definition
hierarchy
Generally speaking, hierarchy refers to an organizational structure in which items are ranked in a specific manner, usually ...
See complete definition
A charge-coupled device (CCD) is a light-sensitive integrated circuit that captures images by converting photons to electrons . A CCD sensor breaks the image elements into pixels . Each pixel is converted into an electrical charge whose intensity is related to the intensity of light captured by that pixel.
For many years, CCDs were the sensors of choice in a wide range of devices, but they're steadily being replaced by image sensors based on complementary metal-oxide-semiconductor ( CMOS ) technology.
The CCD was invented in 1969 at Bell Labs -- now part of Nokia -- by George Smith and Willard Boyle. However, the researcher's efforts were focused primarily on computer memory , and it wasn't until the 1970s that Michael F. Tompsett, also with Bell Labs, refined the CCD's design to better accommodate imaging.
After that, the CCD continued to be improved by Tompsett and other researchers, leading to enhancements in light sensitivity and overall image quality. The CCD soon becoming the primary technology used for digital imagery .
Small, light-sensitive areas are etched into a silicon surface to create an array of pixels that collect the photons and generate electrons. The number of electrons in each pixel is directly proportional to the intensity of light captured by the pixel. After all the electrons have been generated, they undergo a shifting process that moves them toward an output node, where they're amplified and converted to voltage .
In the past, CCDs could deliver much better quality images than other types of sensors, including those based on CMOS technology. As a result, they were used in a wide range of devices, including scanners , bar code readers , microscopes, medical equipment and astronomical telescopes. The devices also found use in machine vision for robots, optical character recognition ( OCR ), processing satellite photographs and radar imagery, especially in meteorology.
In addition, CCDs were used in digital cameras to deliver better resolution than older technologies. By 2010, digital cameras could produce images with over one million pixels, yet they sold for under $1,000. The term megapixel was coined in reference to such cameras.
Despite the early successes of CCDs, CMOS sensors have been gaining favor across the industry, and they're now used extensively in consumer products for capturing images. CMOS sensors are easier and cheaper to manufacturer than CCD sensors. They also use less energy and produce less heat.
Even so, CMOS sensors have a reputation for being more susceptible to image noise , which can impact quality and resolution. But their quality has improved significantly in recent years, and CMOS sensors now dominate the image sensor market.
Despite the proliferation of CMOS sensors, CCD sensors are still used for applications that demand precision and a high degree of sensitivity. For example, CCD sensors continue to be used in medical, scientific and industrial equipment. Even the Hubble Space Telescope sports a CCD sensor. But the momentum is clearly behind CMOS, and the future of CCD remains unclear.
ISO/TS 22317:2021 is a useful tool for BCDR practitioners and other personnel tasked with executing a BIA, which can often be a ...
AI is a helpful way to take a different look at risk assessment and disaster recovery. AI sifts through massive amounts of risk ...
Budgeting for disaster recovery does not need to be difficult. With detailed guidance from our free budget template, IT ...
Continuous data protection can eliminate your backup window. However, there are many issues to consider, including application ...
Folio Photonics has increased the layers of optical disk, lowering the cost and increasing the capacity while delivering higher ...
To help customers avoid costly egress and geo-replication fees, XenData has introduced a new tape archive appliance for local ...
File classification with File Server Resource Manager enables admins to classify and organize data. This tutorial shows how to ...
Even with government money soon headed their way, top-tier semiconductor companies will still have to deal with a range of ...
Blockchain has been a significant contributor to the global chip shortage. Explore the role this rising technology has played.
All Rights Reserved,
Copyright 2000 - 2022 , TechTarget
Privacy Policy
Cookie Preferences
Do Not Sell My Personal Info
Home
Microscopy Basics
Techniques
Confocal
DIC
Fluorescence
Light Sheet
Multiphoton
Phase Contrast
Polarized Light
Super-Resolution
Stereomicroscopy
Applications
Live-Cell Imaging
Förster Resonance Energy Transfer (FRET)
Fluorescence in situ Hybridization (FISH)
Digital Imaging
Tutorials
Galleries
Cell Motility
Confocal
Differential Interference Contrast (DIC)
Fluorescence
Human Pathology
Phase Contrast
Polarized Light
Stereomicroscopy
Nikon’s Small World
Digital Imaging
Museum
Digital camera systems , incorporating a variety of charge-coupled device ( CCD )
detector configurations, are by far the most common image capture
technology employed in modern optical microscopy. Until recently,
specialized conventional film cameras were generally used to record
images observed in the microscope. This traditional method, relying on
the photon-sensitivity of silver-based photographic film, involves
temporary storage of a latent image in the form of photochemical
reaction sites in the exposed film, which only becomes visible in the
film emulsion layers after chemical processing (development).
Digital cameras replace the sensitized film with a CCD photon
detector, a thin silicon wafer divided into a geometrically regular
array of thousands or millions of light-sensitive regions that capture
and store image information in the form of localized electrical charge
that varies with incident light intensity. The variable electronic
signal associated with each picture element (pixel) of the detector is
read out very rapidly as an intensity value for the corresponding image
location, and following digitization of the values, the image can be
reconstructed and displayed on a computer monitor virtually
instantaneously.
Several digital camera systems designed specifically for optical
microscopy are illustrated in Figure 1 . The Nikon Digital Eclipse
DXM1200 provides high quality photo-realistic digital images at
resolutions ranging up to 12 million pixels with low noise, superb color
rendition, and high sensitivity. The camera is controlled by software
that allows the microscopist a great deal of latitude in collecting,
organizing, and correcting digital images. Live color monitoring on the
supporting computer screen at 12 frames per second enables easy
focusing of images, which can be saved with a choice of three formats: JPG , TIF , and BMP for greater versatility.
The DS-5M-L1 Digital Sight camera system ( Figure 1 ) is Nikon's
innovative digital imaging system for microscopy that emphasizes the
ease and efficiency of an all-in-one concept, incorporating a built-in
LCD monitor in a stand-alone control unit. The system optimizes the
capture of high-resolution images up to 5 megapixels through
straightforward menus and pre-programmed imaging modes for different
observation methods. The stand-alone design offers the advantage of
independent operation including image storage to a CompactFlash Card
housed in the control/monitor unit, but has the versatility of full
network capabilities if desired. Connection is possible to PCs through a
USB interface, and to local area networks or the Internet via Ethernet
port. Web browser support is available for live image viewing and remote
camera control, and the camera control unit supports HTTP, Telnet, FTP
server/client, and is DHCP compatible. The camera systems illustrated
in Figure 1 represent the advanced technology currently available for
digital imaging with the optical microscope.
Perhaps the single most significant advantage of digital image
capture in optical microscopy, as exemplified by CCD camera systems, is
the possibility for the microscopist to immediately determine whether a
desired image has been successfully recorded. This capability is
especially valuable considering the experimental complexities of many
imaging situations and the transient nature of processes that are
commonly investigated. Although the charge-coupled device detector
functions in an equivalent role to that of film, it has a number of
superior attributes for imaging in many applications. Scientific-grade
CCD cameras exhibit extraordinary dynamic range, spatial resolution,
spectral bandwidth, and acquisition speed. Considering the high light
sensitivity and light collection efficiency of some CCD systems, a film
speed rating of approximately ISO 100,000 would be required to produce
images of comparable signal-to-noise ratio ( SNR ). The spatial
resolution of current CCDs is similar to that of film, while their
resolution of light intensity is one or two orders of magnitude better
than that achieved by film or video cameras. Traditional photographic
films exhibit no sensitivity at wavelengths exceeding 650 nanometers in
contrast to high-performance CCD sensors, which often have significant
quantum efficiency into the near infrared spectral region. The linear
response of CCD cameras over a wide range of light intensities
contributes to the superior performance, and gives such systems
quantitative capabilities as imaging spectrophotometers.
A CCD imager consists of a large number of light-sensing elements
arranged in a two-dimensional array on a thin silicon substrate. The
semiconductor properties of silicon allow the CCD chip to trap and hold
photon-induced charge carriers under appropriate electrical bias
conditions. Individual picture elements, or pixels, are defined in the
silicon matrix by an orthogonal grid of narrow transparent
current-carrying electrode strips, or gates , deposited on the chip. The fundamental light-sensing unit of the CCD is a metal oxide semiconductor ( MOS )
capacitor operated as a photodiode and storage device. A single MOS
device of this type is illustrated in Figure 2 , with reverse bias
operation causing negatively charged electrons to migrate to an area
underneath the positively charged gate electrode. Electrons liberated
by photon interaction are stored in the depletion region up to the full well
reservoir capacity. When multiple detector structures are assembled
into a complete CCD, individual sensing elements in the array are
segregated in one dimension by voltages applied to the surface
electrodes and are electrically isolated from their neighbors in the
other direction by insulating barriers, or channel stops , within the silicon substrate.
The light-sensing photodiode elements of the CCD respond to incident
photons by absorbing much of their energy, resulting in liberation of
electrons, and the formation of corresponding electron-deficient sites
(holes) within the silicon crystal lattice. One electron-hole pair is
generated from each absorbed photon, and the resulting charge that
accumulates in each pixel is linearly proportional to the number of
incident photons. External voltages applied to each pixel's electrodes
control the storage and movement of charges accumulated during a
specified time interval. Initially, each pixel in the sensor array
functions as a potential well to store the charge during
collection, and although either negatively charged electrons or
positively charged holes can be accumulated (depending on the CCD
design), the charge entities generated by incident light are usually
referred to as photoelectrons . This discussion considers
electrons to be the charge carriers. These photoelectrons can be
accumulated and stored for long periods of time before being read from
the chip by the camera electronics as one stage of the imaging process.
Image generation with a CCD camera can be divided into four primary
stages or functions: charge generation through photon interaction with
the device's photosensitive region, collection and storage of the
liberated charge, charge transfer, and charge measurement. During the
first stage, electrons and holes are generated in response to incident
photons in the depletion region of the MOS capacitor structure, and
liberated electrons migrate into a potential well formed beneath an
adjacent positively-biased gate electrode. The system of aluminum or
polysilicon surface gate electrodes overlie, but are separated from,
charge carrying channels that are buried within a layer of insulating
silicon dioxide placed between the gate structure and the silicon
substrate. Utilization of polysilicon as an electrode material provides
transparency to incident wavelengths longer than approximately 400
nanometers and increases the proportion of surface area of the device
that is available for light collection. Electrons generated in the
depletion region are initially collected into electrically positive
potential wells associated with each pixel. During readout, the
collected charge is subsequently shifted along the transfer channels
under the influence of voltages applied to the gate structure. Figure 3
illustrates the electrode structure defining an individual CCD sense
element.
In general, the stored charge is linearly proportional to the light
flux incident on a sensor pixel up to the capacity of the well;
consequently this full-well capacity ( FWC ) determines the
maximum signal that can be sensed in the pixel, and is a primary factor
affecting the CCD's dynamic range. The charge capacity of a CCD
potential well is largely a function of the physical size of the
individual pixel. Since first introduced commercially, CCDs have
typically been configured with square pixels assembled into rectangular
area arrays, with an aspect ratio of 4:3 being most common. Figure 4
presents typical dimensions of several of the most common sensor formats
in current use, with their size designations in inches according to a
historical convention that relates CCD sizes to vidicon tube diameters.
The rectangular geometry and common dimensions of CCDs result from
their early competition with vidicon tube cameras, which required the
solid-state sensors to produce an electronic signal output that
conformed to the prevailing video standards at the time. Note that the
"inch" designations do not correspond directly to any of the CCD
dimensions, but represent the size of the rectangular area scanned in
the corresponding round vidicon tube. A designated "1-inch" CCD has a
diagonal of 16 millimeters and sensor dimensions of 9.6 x 12.8
millimeters, derived from the scanned area of a 1-inch vidicon tube with
a 25.4-millimeter outside diameter and an input window approximately 18
millimeters in diameter. Unfortunately, this confusing nomenclature
has persisted, often used in reference to CCD "type" rather than size,
and even includes sensors classified by a combination of fractional and
decimal terms, such as the widely used 1/1.8-inch CCD that is
intermediate in size between 1/2-inch and 2/3-inch devices.
Although consumer cameras continue to primarily employ rectangular
sensors built to one of the "standardized" size formats, it is becoming
increasingly common for scientific-grade cameras to incorporate square
sensor arrays, which better match the circular image field projected in
the microscope. A large range of sensor array sizes are produced, and
individual pixel dimensions vary widely in designs optimized for
different performance parameters. CCDs in the common 2/3-inch format
typically have arrays of 768 x 480 or more diodes and dimensions of 8.8 x
6.6 millimeters (11-millimeter diagonal). The maximum dimension
represented by the diagonal of many sensor arrays is considerably
smaller than the typical microscope field of view, and results in a
highly magnified view of only a portion of the full field. The
increased magnification can be beneficial in some applications, but if
the reduced field of view is an impediment to imaging, demagnifying
intermediate optical components are required. The alternative is use of
a larger CCD that better matches the image field diameter, which ranges
from 18 to 26 millimeters in typical microscope configurations.
An approximation of CCD potential-well storage capacity may be
obtained by multiplying the diode (pixel) area by 1000. A number of
consumer-grade 2/3-inch CCDs, with pixel sizes ranging from 7 to 13
micrometers in size, are capable of storing from 50,000 to 100,000
electrons. Using this approximation strategy, a diode with 10 x 10
micrometer dimensions will have a full-well capacity of approximately
100,000 electrons. For a given CCD size, the design choice regarding
total number of pixels in the array, and consequently their dimensions,
requires a compromise between spatial resolution and pixel charge
capacity. A trend in current consumer devices toward maximizing pixel
count and resolution has resulted in very small diode sizes, with some
of the newer 2/3-inch sensors utilizing pixels less than 3 micrometers
in size.
CCDs designed for scientific imaging have traditionally employed
larger photodiodes than those intended for consumer (especially
video-rate) and industrial applications. Because full-well capacity and
dynamic range are direct functions of diode size, scientific-grade CCDs
used in slow-scan imaging applications have typically employed diodes
as large as 25 x 25 micrometers in order to maximize dynamic range,
sensitivity, and signal-to-noise ratio. Many current high-performance
scientific-grade cameras incorporate design improvements that
Tommy Lee And Pam Anderson Sex Tape
Gladiator36
Tube Pornstars