Black Hole Universe
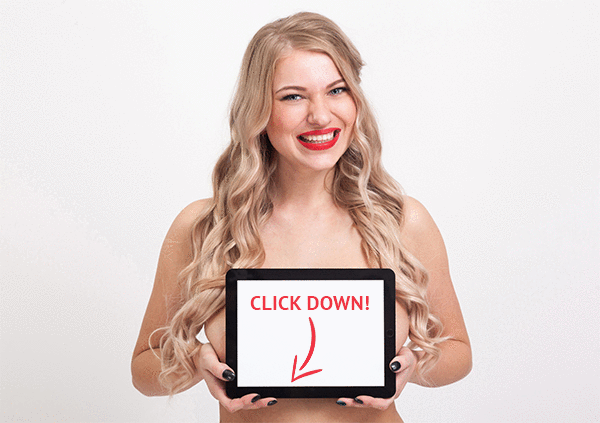
🛑 ALL INFORMATION CLICK HERE 👈🏻👈🏻👈🏻
Black Hole Universe
(opens in new tab)
(opens in new tab)
(opens in new tab)
(opens in new tab)
(opens in new tab)
Contact me with news and offers from other Future brands
Receive email from us on behalf of our trusted partners or sponsors
Space is supported by its audience. When you purchase through links on our site, we may earn an affiliate commission. Here’s why you can trust us .
Our universe might have originated from a black hole that lies within another universe.
The idea centers on how matter and energy falling into a blackhole could in theory come out a " white hole " in another universe.In such a situation, both the black hole and the white hole are mouths of anEinstein-Rosen bridge, popularly known as a wormhole.
With that in mind, theoretical physicist Nikodem Poplawski at Indiana University conjectured that when a black hole forms upon the collapse of a dying star, a universe is born at the same time from the white hole on the other side of the wormhole .
"Our universe could have itself formed from inside a black hole existing inside another universe," he said.
This idea has been suggested before, and now Poplawski has expanded on the thinking.
Although a black hole forming from a star the size of our sun would only be about 2 miles wide, it does not mean that a universe which might originate from a black hole would stay that small.
"Our universe was small a long time ago and expanded," Poplawski said."From the other side, one would not see our expansion." Essentially, a black hole could seem bigger on the "inside" than how it looked to someone outside.
If anyone survived a trip into a black hole and emerged in another universe, "it would be a one-way trip," Poplawski noted. The event horizon of a black hole is ?boundary at which nothing inside can escape.
In theory, black holes do lose mass, however, as Hawking radiation— particles that emerge from the vacuum right next to their event horizons.Black holes that lose more mass than they gain are expected to shrink and ultimately vanish. This does not mean any universe at the other end of the black hole would cease to exist, Poplawski explained. "We would just be disconnected from the other universe," he said.
If our universe was born from a black hole in another universe, it would be impossible to cross its event boundary and see the other side, meaning one cannot prove or disprove this idea that way.
So how might one test this conjecture? One implication of Poplawski's concept is that our universe is "closed."
"In cosmology, there are three models for our universe,"Poplawski explained. "The first is that it's closed, the second is that it's 'open,' and the third is that it's 'flat.'"
If it is closed, and one tries to venture to the edge of our universe , one would eventually loop back to where one started."It would be like walking on the surface of the Earth — if you walk to the east, at some point you come back across the west," Poplawski said.
If our universe is flat, and one tries to venture to its edge, one would never reach it, as it continued on infinitely. The same would hold true if the universe is open, "only it would be 'curved,'" Poplawski said.This means that if two beams of light were shot into space parallel to each other, in a flat universe they would stay parallel, while in an open universe they would actually get farther from each other.
If our universe lies "within" another universe,"the prediction would be that our universe is closed," Poplawski said. "That could maybe tested at some point. If the universe is closed, if one watches the stars, their light would be visible on the opposite side of the sky. If the universe is really big, the light from those stars might not have had time to do that yet, but maybe at some point we could see this."
Also, black holes rotate, evidence suggests, and if our universe's parent black hole was spinning, "then one direction might be preferred in our universe, evidence we could see at large scales," Poplawski said.
If our universe was born from a black hole, it could help solve the mystery of what came before the BigBang and whether our universe was closed, flat or open.
It could also help scientists avoid problems associated with singularities, or infinitely small points with infinite density. In theory, the universe was born from a singularity, and every black hole encloses a singularity. However, singularities are big headaches for theoretical physicists, since the laws of physics break down around them.
"In my model, when the universe is born, there is no problem of having a singularity initially — instead of being infinitely small, it had the radius of its parent black hole," Poplawski said. "Also, instead of black holes ending up with singularities, this model suggests they would end up with universes."
One hole in this idea is one that turns up whenever wormholes are discussed — exotic matter .
In principle, all wormholes are unstable, closing the instant they open. The only way to keep them open is with an exotic form of matter with so-called "negative energy density." Such exotic matter has bizarre properties, including moving in the opposite direction of normal matter when pushed. No one knows if such matter actually exists.
"It is possible that when black holes form, matter can undergo a phase transition to become exotic matter, to allow this initial expansion of a universe inside the black hole," Poplawski suggested.
Also, while this might explain how our universe was born from a black hole, it does not explain how the universe that black hole is from came to be. "So was that universe born from a black hole in another universe, and so on?" Poplawski noted. "It's a problem of an infinite number of universes. That's a very reasonable criticism."
Poplawski will detail his research April 12 in the journal edition of Physics LettersB .
Join our Space Forums to keep talking space on the latest missions, night sky and more! And if you have a news tip, correction or comment, let us know at: community@space.com.
Charles Q. Choi is a contributing writer for Space.com and Live Science. He covers all things human origins and astronomy as well as physics, animals and general science topics. Charles has a Master of Arts degree from the University of Missouri-Columbia, School of Journalism and a Bachelor of Arts degree from the University of South Florida. Charles has visited every continent on Earth, drinking rancid yak butter tea in Lhasa, snorkeling with sea lions in the Galapagos and even climbing an iceberg in Antarctica. Visit him at http://www.sciwriter.us
Strange molecules swirl around supermassive black holes, James Webb Space Telescope finds
Pictures from space! Our image of the day
Fallen Arecibo Observatory telescope won't be rebuilt despite scientists' hopes
Get breaking space news and the latest updates on rocket launches, skywatching events and more!
Thank you for signing up to Space. You will receive a verification email shortly.
There was a problem. Please refresh the page and try again.
Space is part of Future US Inc, an international media group and leading digital publisher. Visit our corporate site (opens in new tab) .
©
Future US, Inc. Full 7th Floor, 130 West 42nd Street,
New York,
NY 10036.
Black Holes
Basics
Types
Anatomy
Gallery
Basics
Types
Anatomy
Gallery
This simulation shows a black hole distorting a starry background. Credit: NASA’s Goddard Space Flight Center; background, ESA/Gaia/DPAC more
NASA’s newest X-ray eyes are open and ready for discovery.
NASA’s IXPE mission launched Thursday, December 9, 2021, NASA’s Kennedy Space Center in Florida.
NASA’s Roman will use its microlensing survey to provide the best opportunity yet to definitively detect solitary small black holes.
Black holes are some of the most bizarre and fascinating objects in the cosmos – but they're invisible!
For the first time, scientists mapped out the invisible magnetic fields pulsing through Centaurus A using infrared light.
An international team of astronomers discovered a cosmic equivalent to Yellow Stone's Old Faithful geyser.
After more than 16 years studying the universe in infrared light, NASA's Spitzer mission has come to an end.
For the first time, NASA’s TESS watched a black hole tear apart a star.
NuSTAR’s observations of an ultraluminous X-ray source offer possible explanations for these mysterious objects.
Since its launch, NASA’s Spitzer has lifted the veil on the wonders of the cosmos using infrared light.
By combining NASA Chandra data with the EHT image, scientists can learn more about the giant black hole M87* and its behavior.
Scientists charted the environment surrounding a stellar-mass black hole using NASA’s NICER.
Did you know our Milky Way galaxy is blowing bubbles? Two of them, each 25,000 light-years tall!
For the first time, scientists using NASA’s Fermi found the source of a high-energy neutrino from outside our galaxy.
NASA’s Chandra data were critical in determining if a neutron star merger created a heavier neutron star or black hole.
NuSTAR identified two gas-enshrouded supermassive black holes at the centers of nearby galaxies.
These data from NASA’s Chandra gave astronomers a look at the growth of black holes over billions of years.
NASA's Swift and NuSTAR caught a supermassive black hole in the midst of a giant eruption of X-ray light.
Astronomers used NASA’s Swift satellite to detect the awakening of a distant galaxy's dormant black hole.
NASA's Fermi unveiled a previously unseen structure centered in the Milky Way.
NASA scientists have, for the first time, detected and pinned down the location of a short gamma-ray burst.
NASA Chandra data has shown ripples in the hot gas that fills the Perseus cluster are sound waves.
Hubble found seemingly conclusive evidence for a massive black hole in the center of the giant elliptical galaxy M87.
Basics
Building Blocks
Forces
Gallery
News & Features
Resources
Glossary
This site is maintained by the Astrophysics Communications teams at NASA's Goddard Space Flight Center and NASA's Jet Propulsion Laboratory for NASA's Science Mission Directorate.
From stellar-mass black holes with a few times our Sun’s mass to supermassive monsters weighing up to billions more, black holes' makeup is essentially identical. As weird as black holes seem, they possess only three properties: mass, spin, and electrical charge. The list below describes many of the features common to the black holes astronomers are studying.
Tap a plus icon above or click the arrows below to continue.
Tap a plus icon above or click the arrows below to continue.
Tap a plus icon above or click the arrows below to continue.
Tap a plus icon above or click the arrows below to continue.
Tap a plus icon above or click the arrows below to continue.
Tap a plus icon above or click the arrows below to continue.
Tap a plus icon above or click the arrows below to continue.
Tap a plus icon above or click the arrows below to continue.
Tap a plus icon above or click the arrows below to continue.
Background image credit: NASA’s Goddard Space Flight Center/Jeremy Schnittman
This is what makes a black hole black. We can think of the event horizon as the black hole’s surface. Inside this boundary, the velocity needed to escape the black hole exceeds the speed of light, which is as fast as anything can go. So whatever passes into the event horizon is doomed to stay inside it – even light. Because light can’t escape, black holes themselves neither emit nor reflect it, and nothing about what happens within them can reach an outside observer. But astronomers can observe black holes thanks to light emitted by surrounding matter that hasn’t yet dipped into the event horizon.
The main light source from a black hole is a structure called an accretion disk. Black holes grow by consuming matter, a process scientists call accretion, and by merging with other black holes. A stellar-mass black hole paired with a star may pull gas from it, and a supermassive black hole does the same from stars that stray too close. The gas settles into a hot, bright, rapidly spinning disk. Matter gradually works its way from the outer part of the disk to its inner edge, where it falls into the event horizon. Isolated black holes that have consumed the matter surrounding them do not possess an accretion disk and can be very difficult to find and study.
If we could see it up close, we’d find that the accretion disk has a funny shape when viewed from most angles. This is because the black hole’s gravitational field warps space-time, the fabric of the universe, and light must follow this distorted path. Astronomers call this process gravitational lensing. Light coming to us from the top of the disk behind the black hole appears to form into a hump above it. Light from beneath the far side of the disk takes a different path, creating another hump below. The humps’ sizes and shapes change as we view them from different angles, and we see no humps at all when seeing the disk exactly face on.
The event horizon captures any light passing through it, and the distorted space-time around it causes light to be redirected through gravitational lensing. These two effects produce a dark zone that astronomers refer to as the event horizon shadow, which is roughly twice as big as the black hole’s actual surface.
From every viewing angle, thin rings of light appear at the edge of the black hole shadow. These rings are really multiple, highly distorted images of the accretion disk. Here, light from the disk actually orbits the black hole multiple times before escaping to us. Rings closer to the black hole become thinner and fainter.
Viewed from most angles, one side of the accretion disk appears brighter than the other. Near the black hole, the disk spins so fast that an effect of Einstein’s theory of relativity becomes apparent. Light streaming from the part of the disk spinning toward us becomes brighter and bluer, while light from the side the disk rotating away from us becomes dimmer and redder. This is the optical equivalent of an everyday acoustic phenomenon, where the pitch and volume of a sound – such as a siren – rise and fall as the source approaches and passes by. The black hole’s particle jets show off this effect even more dramatically.
It’s been called one of the most extreme physical environments in the universe. Strong magnetic fields threading the inner accretion disk extend out of it, creating a tenuous, turbulent, billion-degree cloud. Particles in the corona orbit the black hole at velocities approaching the speed of light. It’s a source of X-rays with much higher energies than those emanating from the accretion disk, but astronomers are still trying to figure out its extent, shape, and other characteristics.
In black holes of all sizes, something strange can occur near the inner edge of the accretion disk. A small amount of material heading toward the black hole may suddenly become rerouted into a pair of jets that blast away from it in opposite directions. These jets fire out particles at close to the speed of light, but astronomers don’t fully understand how they work. The jets from supermassive black holes – the type found in the centers of most big galaxies – can reach lengths of hundreds of thousands of light-years. In cases where the jets happen to angle into our line of sight, we may only easily detect the one firing toward us due to Doppler beaming. This process makes the near jet considerably brighter, but greatly dims the rear jet.
General relativity predicts that the very center of a black hole contains a point where matter is crushed to infinite density. It’s the final destination for anything falling into the event horizon. The singularity may be either a physical structure or a purely mathematical one, but right now astronomers don’t know which is true. The prediction of a singularity may signal the limits of relativity, where quantum effects not included in the theory become important in a more complete description of gravity.
Director, NASA Astrophysics Division:
Dr. Mark Clampin
NASA Officials:
Dr. Amber Straughn and Anya Biferno
Site Managers:
Chelsea Gohd and Jeanette Kazmierczak
Social Media Lead:
Sara Mitchell
From Wikipedia, the free encyclopedia
Astronomical object that has a no-return boundary
G
μ
ν
+
Λ
g
μ
ν
=
κ
T
μ
ν
{\displaystyle G_{\mu \nu }+\Lambda g_{\mu \nu }={\kappa }T_{\mu \nu }}
Simple illustration of a non-spinning black hole
Artistic depiction of a black hole and its features
Far away from the black hole, a particle can move in any direction, as illustrated by the set of arrows. It is restricted only by the speed of light.
Closer to the black hole, spacetime starts to deform. There are more paths going towards the black hole than paths moving away. [Note 3]
Inside of the event horizon, all paths bring the particle closer to the centre of the black hole. It is no longer possible for the particle to escape.
The formula for the Bekenstein–Hawking entropy ( S ) of a black hole, which depends on the area of the black hole ( A ). The constants are the speed of light ( c ), the Boltzmann constant ( k ), Newton's constant ( G ), and the reduced Planck constant ( ħ ). In Planck units, this reduces to S = A / 4 .
^ The value of cJ/GM 2 can exceed 1 for objects other than black holes. The largest value known for a neutron star is ≤ 0.4, and commonly used equations of state would limit that value to < 0.7. [76]
^ The (outer) event horizon radius scales as:
M
+
M
2
−
(
J
/
M
)
2
−
Q
2
.
{\displaystyle M+{\sqrt {M^{2}-{(J/M)}^{2}-Q^{2}}}.}
^ The set of possible paths, or more accurately the future light cone containing all possible world lines (in this diagram the light cone is represented by the V-shaped region bounded by arrows representing light ray world lines), is tilted in this way in Eddington–Finkelstein coordinates (the diagram is a "cartoon" version of an Eddington–Finkelstein coordinate diagram), but in other coordinates the light cones are not tilted in this way, for example in Schwarzschild coordinates they simply narrow without tilting as one approaches the event horizon, and in Kruskal–Szekeres coordinates the light cones do not change shape or orientation at all. [79]
^ This is true only for four-dimensional spacetimes. In higher dimensions more complicated horizon topologies like a black ring are possible. [91] [92]
^ In pa
Mistress Gagged
Slim Hard Sloppy Gagging
Hd1080 Porno Hair