Black Hole Rubicon
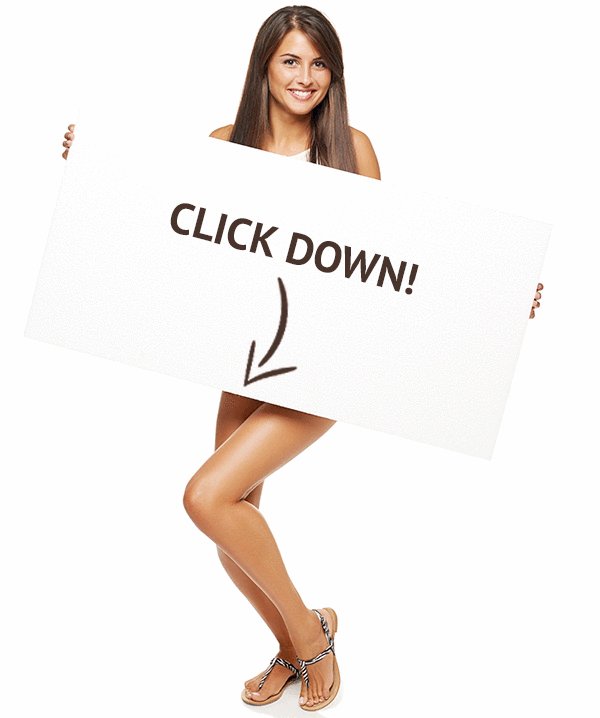
🔞 ALL INFORMATION CLICK HERE 👈🏻👈🏻👈🏻
Black Hole Rubicon
By Steve Nadis Dec 20, 2011 12:00 AM
(Credit: Andrew Hamilton/Univ. of Colorado, Boulder; Axel Mellinger/Central Michigan Univ.; John Hawley/Univ. of Virginia)
Sign up for our email newsletter for the latest science news
(Credit: Andrew Hamilton/Univ. of Colorado, Boulder; Axel Mellinger/Central Michigan Univ.; John Hawley/Univ. of Virginia)
(Credit: Andrew Hamilton/Univ. of Colorado, Boulder; Axel Mellinger/Central Michigan Univ.; John Hawley/Univ. of Virginia)
Want More? Get unlimited access for as low as $1.99/month
Copyright © 2022 Kalmbach Media Co.
It is late December and snow is swirling as Andrew Hamilton coasts up to his office at the University of Colorado’s Boulder campus, in the foothills of the Rockies. On a blustery day like today, most of his colleagues arrive in SUVs or at least in cars shod with all-season tires. Hamilton rides in on his Cannondale mountain bike.
Following his own path is not just a pastime to Hamilton, it is the essence of his career. For 15 years the astrophysicist has ventured nearly alone into the darkest, most impenetrable part of the universe: the inside of a black hole. “I’m not religious, but I share with religious people a desire to understand the truth about our universe. I’m focused on attaining a complete understanding of the interior of black holes,” he says, his British accent adding solemnity and power to his words. That quest has been called mad or just plain futile by colleagues who insist that the inner structure of the black hole is so extreme that it lies not only beyond exploration but beyond comprehension. Hamilton, an athletic 59-year-old with a mane of sandy blond hair, brushes such doubt away. “I don’t necessarily avoid things others consider crazy, or I never would have gotten started in this black hole business. I’m a guy who likes adversity. I like to struggle. It’s fun to try to beat the odds.”
Black holes are massive objects that have collapsed in on themselves, creating a gravitational suction so intense that their insides become cut off from the rest of the universe. A black hole’s outer boundary, known as the event horizon , is a point of no return. Once trapped inside, nothing—not even light—can escape. At the center is a core, known as a singularity, that is infinitely small and dense, an affront to all known laws of physics. Since no energy, and hence no information, can ever leave that dark place, it seems quixotic to try peering inside. As with Las Vegas, what happens in a black hole stays in a black hole.
Where other scientists see the end point of scientific inquiry, Hamilton sees the beginning, an entrée to an extraordinary and unexplored terrain. He pictures a waterfall of space and time pouring over the event horizon to an inner zone where “all the light and material that ever fell into the black hole piles up in a tremendous collision, generating a maelstrom of energy and an infinitely bright, blinding flash of light.” Then he jumps in his barrel and takes the plunge.
Hamilton’s work has the flavor of a charming personal obsession, but it also has huge implications. Black holes are connected to some of the most basic phenomena in our universe. They may have been among the universe’s earliest structures, influencing the formation and evolution of galaxies like our own. Almost every large galaxy still houses a monster black hole, up to billions of times the mass of our sun, at its center. Much smaller black holes (typically weighing several times as much as the sun) result from the explosive death of ultrabright stars, so these dark objects reveal secrets about the stellar life cycle. Our galaxy alone may contain 100 million such objects. And because of parallels between black holes and the Big Bang, black holes might help explain how the universe was formed. In some exotic physics theories, they could even house wormholes—hypothetical shortcuts across space and time —or function as nurseries where other universes are born.
“Black holes are vaults harboring some of the most fundamental truths of the cosmos,” Hamilton says. By applying the laws of physics, he is trying to unlock the secrets inside.
Hamilton began his journey to the heart of darkness somewhat unwittingly as he carried out the most straightforward part of his job: teaching undergraduate astronomy at the University of Colorado. It was 1996, and Hamilton had asked some of his students to make a black hole show for the university’s Fiske Planetarium. They created a variety of short presentations, highlighting various aspects of these astrophysical objects. For the finale of their presentation, the students tacked on a 40-second clip from Stargate , a 1994 movie in which an Egyptologist (James Spader) pushes through the fluidlike surface of the “ stargate ,” a 20-foot-wide, hieroglyph-adorned ring providing passage to a parallel world. Hamilton’s heart sank. The movie’s set pieces bore no relationship to real physics. But when Spader passed through the wormhole, encountering a multicolored, psychedelic display of geometric patterns and streaming lights, the students cheered and Hamilton began to change his mind. Science-based visualizations could be an effective way to teach people about black holes—“maybe the best way,” he thought. He vowed to create his own depiction of the descent through a black hole, one based not on Hollywood sleight of hand but on the best physics he could find.
Hamilton did not know much about black holes back then, but he had a lot of related expertise to draw on. For years he had been studying the origin of the universe, working backward in time from the current arrangement of galaxies to infer conditions in the era immediately after the Big Bang. A black hole, Hamilton realized, could be thought of as a kind of Big Bang in reverse. Instead of exploding outward from an infinitesimally small point, spewing matter and energy and space to create the cosmos, a black hole pulls everything inward toward a single, dense point. Whether in a black hole or in the Big Bang, the ultimate point—the singularity—is where everything started and where it all might end. One important difference, though, is that the Big Bang occurred some 13.7 billion years ago. Black holes are abundant and practically ubiquitous, and they are here right now.
That link between black holes and the Big Bang hooks into one of the most pressing mysteries of modern physics: reconciling the laws that govern the large-scale world we inhabit and those governing the invisible, subatomic realm. Planets, stars, and galaxies seem to follow the rules of Albert Einstein’s general theory of relativity , which describes gravity and the curvature of space-time. (According to Einstein, mass bends both space and time, causing objects to fall toward it and creating the phenomenon that we perceive as gravity.) Small-scale objects like atoms and electrons, on the other hand, seem to follow the very different laws of quantum mechanics. The two sets of rules must ultimately mesh, but physicists are still trying to figure out how. For the Big Bang and the black hole, the disconnect is especially obvious because they occupy both the very big and the very small ends of the scale as they evolve. “I was interested in general relativity as a graduate student,” Hamilton says. “This project allowed me to work on general relativity and make movies at the same time.”
Despite the magnitude of the task, Hamilton began modestly. He took the known attributes of black holes and plugged them into a basic computer graphics program. All it involved was applying Einstein’s relativity equations, which describe how light rays would bend as they approach a black hole. Hamilton’s first, simple movies were broad and cartoonish, but they served their purpose: showing how different kinds of black holes might look as you approached them from the outside and then ventured in. In one animation, the observer flew by a star system and plunged across a black hole's event horizon, represented by a spherical red grid. Another movie offered a glimpse of an alternate universe, shown in pink, before the observer met his end at the singularity. In a third, the event horizon split in two as the observer entered the interior—a bizarre effect (later validated by Hamilton) that initially convinced some critics that these simulations must be flawed.
Things took off from there in ways that Hamilton never planned. In 2001 executives from the Denver Museum of Nature and Science visited Hamilton’s department with a proposition. They were building a new planetarium with a state-of-the-art digital projection system, and they needed help developing eye-popping shows. “This was a tremendous opportunity to put black holes on domes and take my visualizations to the next level,” Hamilton says.
He was so excited that he went on a yearlong sabbatical and spent his time developing
visualization software far more powerful than the off-the-shelf program he had been using. He started with an interface designed for creating video games. Then he incorporated existing solutions to Einstein’s equations to produce black hole simulations that “could show what it actually would look like if you were there.” These virtual black holes were more complex and realistic than his original renderings because users could adjust the size of the hole, choose what kind of object to feed it, and change the viewing angle to watch the action unfold. His final software package had more than 100,000 lines of code—as complex as the modeling programs scientists use on the world’s fastest supercomputers.
Hamilton’s black hole simulator attracted attention, and in 2002 he was invited to collaborate on a Nova documentary about black holes. That is when Hamilton had to face the painful truth that all his visualizations to date had been based on calculations done by others. Despite his new role as scientific director for a television program explaining what would happen if someone fell into a black hole, he had not yet done the heavy lifting by working through Einstein’s equations to describe gravity and the curvature of space-time in realistic environments. Astronomers knew, for example, that in the centers of some galaxies, black holes suck up vast amounts of gas—the equivalent of our whole solar system—every year. Standard theory held that a black hole’s intense gravity pulled all that material toward the singularity in the center, where space and time as we knew them came to an end. But was that really true? What happened to all that cosmic detritus on its journey to the core?
Addressing such questions meant Hamilton had to take another leap, grappling with the equations of relativity in detail. In Einstein’s geometric conception of gravity, a massive body like the sun dents the fabric of space-time, much as a large person deforms the surface of a trampoline. Earth follows the curved shape of the warped space around the sun, which is why it moves in a circular orbit; this description has been experimentally verified to high precision.
Ten linked equations— Einstein’s field equations —describe precisely how space-time is curved for any given distribution of matter and energy, even for something as extreme as a black hole. Hamilton knew he was in for a bumpy ride, however. Relativity is confusing enough for conventional objects. It is far stranger for a black hole because such an object does not merely dent space-time; it creates a discontinuity, a bottomless pit in the middle of an otherwise smooth fabric.
Hamilton tried to make the problem more manageable by looking at black holes from a different perspective. He proposed a new analogy to describe what happens when something, or someone, approaches a black hole’s event horizon, likening it to a waterfall crashing into an abyss . A fish can swim near the edge and safely slip away—unless it gets too close, in which case it will be dragged over the precipice no matter how hard it resists. Similarly, any object or even any kind of energy is swept across the event horizon by a “waterfall” of space that is constantly cascading into the black hole. If a flashlight sailed over the edge of that metaphorical waterfall, not only the flashlight but also its light beam would be pulled in. Hamilton describes a black hole as “a place where space is falling faster than light.” (No object can move through space faster than light, but there is no restriction on how quickly space itself can move.)
When Hamilton had begun thinking about black holes eight years earlier, he started with a simplistic description called a Schwarzschild black hole, a make-believe object that has no charge or spin. But now, hoping to publish in a serious scientific journal, he considered a more complex, albeit still idealized, version: a nonrotating black hole that has an electric charge. Black holes in nature will not collect appreciable charge, but a charged black hole turns out to be a convenient analog for studying a more realistic rotating black hole.
Hamilton also recognized that real black holes do not exist in isolation, so he used the computer model to feed his black hole. Atoms, light, dark matter, the kitchen sink—it was all grist for the ravenous void. Each step toward reality made the model far more intricate. “If there’s nothing around the black hole, you get standard solutions,” Hamilton says. “But if you introduce even the tiniest amount of matter or energy, the whole house of cards collapses.”
The more Hamilton worked with his computer models, the more he realized just how strange the interior of a black hole is. A charged black hole actually has a secondary boundary—an inner horizon—inside the main event horizon that defines the hole’s outer limit. Physics legend Roger Penrose had been the first person to show that something bizarre must happen at that inner horizon, because all the matter and energy falling into a black hole piles up there. Now Hamilton wanted to visualize those conditions in a physically meaningful way. So he kept plugging his increasingly realistic assumptions into his computer and set about solving Einstein’s field equations again and again, each time marveling at the results that came pouring out.
The inner horizon, Hamilton discovered, may be the most energetic and violently unstable place in the universe. Building on the groundbreaking work of physicists Eric Poisson of the University of Guelph in Ontario and Werner Israel of the University of Victoria in British Columbia, he describes the conditions at the inner horizon as an “inflationary instability.” It is inflationary because everything—mass, energy, pressure—keeps growing exponentially. And it is unstable because, according to Hamilton’s calculations, the surface—the inner horizon—cannot sustain itself and must ultimately collapse.
Then things got stranger still. Continuing his quest for realism, Hamilton considered the case of a black hole that rotates (as every known object in the universe, and perhaps the universe itself, does) and plugged it into his computer models. As a test he dropped imaginary particles into his black hole to see what would happen. When a particle falls into a black hole and approaches the inner horizon, he found, it is diverted into one of two narrowly focused, laserlike beams. If the particle enters in the direction opposite that of the black hole’s rotation, it will join an “ingoing beam” that has positive energy and moves forward in time. But here is the real brainteaser: If the particle enters in the same direction as the black hole’s spin, it joins an “outgoing beam” that has negative energy and moves backward in time.
Trying to make physical sense of these abstract conceptual insights, Hamilton discovered that the inner horizon acts as an astonishingly powerful particle accelerator, shooting the ingoing and outgoing beams past each other at nearly the speed of light. This picture came to him, he says, “simply from staring at the mathematics, from staring at Einstein’s equations. In the inflationary zone, those equations make gravity behave in a very unusual way. The picture is extremely counterintuitive, although one gets used to it eventually.”
A person moving with the outgoing beam (if such a thing were possible) would think he was moving away from the black hole when he was, from an outsider’s perspective, actually being pulled toward its center—the same place that someone traveling with the ingoing beam would inevitably go. Even though both parties are moving toward the center, Hamilton realized, the extreme curvature of space-time would cause them to feel like they were falling in different directions.
This particle accelerator has another peculiar attribute: Once started, it never stops. The faster the streams move, the more energy there is; the more energy there is, the more gravity there is, and the faster the particles accelerate. “I look at this and see a machine of remarkable power and deviousness” that gains energy exponentially, yet doesn’t create it from nothing, Hamilton says. “If general relativity is correct, this machine is there and can accelerate particles to enormous energies, reaching—and even exceeding—the Big Bang. So what does nature do with all this energy? Does it all go to waste?”
One potential consequence of the conflagration would be the copious production of mini black holes, each of them starting out far smaller than an atom. The inside of a black hole, then, might contain a dense swarm of smaller black holes. Such mini holes would stay hidden behind the event horizon, but they would affect the inner evolution of their parent. They would combine with each other, trapping stray particles and steadily growing bigger, thereby hastening the collapse of the entire system to a central singularity.
A much more exciting possibility, from Hamilton’s point of view, is that the black hole’s inner accelerator could spawn entire new universes. According to some cosmological models, our universe began as a blip of extreme energy within some other, preexisting universe, which then bubbled off to create a whole reality of its own . Something like this could occur inside a black hole, with a baby universe forming as a tiny bubble at the inner horizon. For a moment this infant would be connected to its “mother” by a kind of umbilical cord, a minuscule wormhole. Then the baby universe would break off to pursue a destiny completely removed from ours. “If there’s anywhere in our universe where baby universes are being created, it’s likely happening inside black holes,” Hamilton says. “And this inflationary zone near the inner horizon is where the process may occur.”
Hamilton’s findings are bad news for anyone hoping to jump into a black hole and tunnel to another place or to one of those other universes. The high-energy particle barrage at the inner horizon would instantly vaporize a human traveler. “The term vaporized, though accurate, is somewhat inadequate simply because we don’t have a word to describe this level of violence,” Hamilton says with a laugh. Actually, a person falling into the inner horizon would meet a worse fate than being reduced to his or her constituent particles. “You’d then take those particles from your body,” Hamilton explains, “and slam them against particles coming the other way at Planck-scale energies”—conditions associated with distances of about 10-35 meter and energies about a quadrillion times what the Large Hadron Collider can achieve. That goes well beyond mere vaporization.
“Perhaps we should call it getting ker-Plancked,” Hamilton jokes.
This year Hamilton is once again on sabbatical. When he is not chipping away at the inner horizon or working on a popular science book about black holes, he spends time with his wife, Catherine, who shares his rugged individualism: She started her own business making and selling cruelty-free stuffed anim
Tranny High Heels
Glamour Models Videos
Gagged Sissy