Black Hole Original
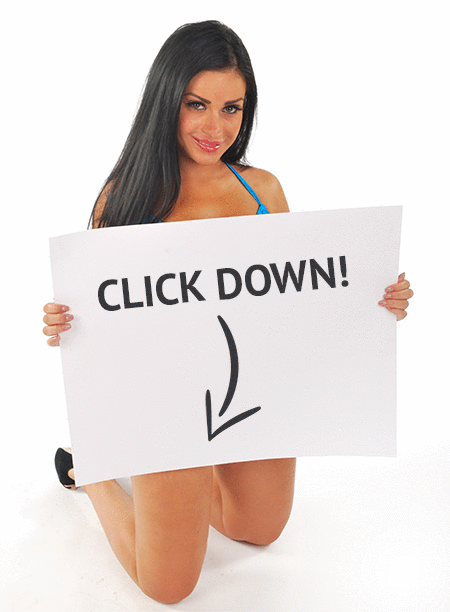
⚡ ALL INFORMATION CLICK HERE 👈🏻👈🏻👈🏻
Black Hole Original
Search By Name
Enter coordinates
Use current location
SUN & MOON
MERCURY & VENUS
MARS, JUPITER & SATURN
US state, Canadian province, or country
Alabama - AL Alaska - AK Arizona - AZ Arkansas - AR California - CA Colorado - CO Connecticut - CT Delaware - DE District of Columbia - DC Florida - FL Georgia - GA Hawaii - HI Idaho - ID Illinois - IL Indiana - IN Iowa - IA Kansas - KS Kentucky - KY Louisiana - LA Maine - ME Maryland - MD Massachusetts - MA Michigan - MI Minnesota - MN Mississippi - MS Missouri - MO Montana - MT Nebraska - NE Nevada - NV New Hampshire - NH New Jersey - NJ New Mexico - NM New York - NY North Carolina - NC North Dakota - ND Ohio - OH Oklahoma - OK Oregon - OR Pennsylvania - PA Puerto Rico - PR Rhode Island - RI South Carolina - SC South Dakota - SD Tennessee - TN Texas - TX Utah - UT Vermont - VT Virginia - VA Washington - WA West Virginia - WV Wisconsin - WI Wyoming - WY --- Alberta - AB British Columbia - BC Manitoba - MB New Brunswick - NB Newfoundland - NF Northwest Territories - NT Nova Scotia - NS Nunavut - NU Ontario - ON Prince Edward Island - PE Quebec - QC Saskatchewan - SK Yukon - YT --- Afghanistan - AFG Albania - ALB Algeria - DZA American Samoa - ASM Andorra - AND Angola - AGO Anguilla - AIA Antarctica - ATA Antigua and Barbuda - ATG Argentina - ARG Armenia - ARM Aruba - ABW Australia - AUS Austria - AUT Azerbaijan - AZE Bahamas - BHS Bahrain - BHR Bangladesh - BGD Barbados - BRB Belarus - BLR Belgium - BEL Belize - BLZ Benin - BEN Bermuda - BMU Bhutan - BTN Bolivia - BOL Bosnia and Herzegovina - BIH Botswana - BWA Bouvet Island - BVT Brazil - BRA British Indian Ocean Territory - IOT Brunei Darussalam - BRN Bulgaria - BGR Burkina Faso - BFA Burundi - BDI Cambodia - KHM Cameroon - CMR Canada - CAN Cape Verde - CPV Cayman Islands - CYM Central African Republic - CAF Chad - TCD Chile - CHL China - CHN Christmas Island - CXR Cocos Islands - CCK Colombia - COL Comoros - COM Cook Islands - COK Costa Rica - CRI Cote d'Ivoire - CIV Croatia - HRV Cuba - CUB Cyprus - CYP Czech Republic - CZE Democratic Republic of the Congo - COD Denmark - DNK Djibouti - DJI Dominica - DMA Dominican Republic - DOM East Timor - TMP Ecuador - ECU Egypt - EGY El Salvador - SLV Equatorial Guinea - GNQ Eritrea - ERI Estonia - EST Ethiopia - ETH Falkland Islands - FLK Faroe Islands - FRO Fiji - FJI Finland - FIN France - FRA France, Metropolitan - FXX French Guiana - GUF French Polynesia - PYF French Southern Territories - ATF Gabon - GAB Gambia - GMB Georgia - GEO Germany - DEU Ghana - GHA Gibraltar - GIB Greece - GRC Greenland - GRL Grenada - GRD Guadeloupe - GLP Guam - GUM Guatemala - GTM Guinea - GIN Guinea-Bissau - GNB Guyana - GUY Haiti - HTI Heard and McDonald Islands - HMD Honduras - HND Hong Kong - HKG Hungary - HUN Iceland - ISL India - IND Indonesia - IDN Iran - IRN Iraq - IRQ Ireland - IRL Isle of Man - IOM Israel - ISR Italy - ITA Jamaica - JAM Japan - JPN Jordan - JOR Kazakhstan - KAZ Kenya - KEN Kiribati - KIR Kuwait - KWT Kyrgyzstan - KGZ Lao - LAO Latvia - LVA Lebanon - LBN Lesotho - LSO Liberia - LBR Libya - LBY Liechtenstein - LIE Lithuania - LTU Luxembourg - LUX Macau - MAC Macedonia - MKD Madagascar - MDG Malawi - MWI Malaysia - MYS Maldives - MDV Mali - MLI Malta - MLT Marshall Islands - MHL Martinique - MTQ Mauritania - MRT Mauritius - MUS Mayotte - MYT Mexico - MEX Micronesia - FSM Moldova - MDA Monaco - MCO Mongolia - MNG Montserrat - MSR Morocco - MAR Mozambique - MOZ Myanmar - MMR Namibia - NAM Nauru - NRU Nepal - NPL Netherlands - NLD Netherlands Antilles - ANT New Caledonia - NCL New Zealand - NZL Nicaragua - NIC Niger - NER Nigeria - NGA Niue - NIU Norfolk Island - NFK North Korea - PRK Northern Mariana Islands - MNP Norway - NOR Oman - OMN Pakistan - PAK Palau - PLW Panama - PAN Papua New Guinea - PNG Paraguay - PRY Peru - PER Philippines - PHL Pitcairn - PCN Poland - POL Portugal - PRT Puerto Rico - PRI Qatar - QAT Republic of the Congo - COG Reunion - REU Romania - ROM Russia - RUS Rwanda - RWA Saint Kitts and Nevis - KNA Saint Lucia - LCA Saint Vincent and the Grenadines - VCT Samoa - WSM San Marino - SMR Sao Tome and Principe - STP Saudi Arabia - SAU Senegal - SEN Seychelles - SYC Sierra Leone - SLE Singapore - SGP Slovakia - SVK Slovenia - SVN Solomon Islands - SLB Somalia - SOM South Africa - ZAF South Georgia and the South Sandwich Islands - SGS South Korea - KOR Spain - ESP Sri Lanka - LKA Saint Helena - SHN St. Pierre and Miquelon - SPM Sudan - SDN Suriname - SUR Svalbard - SJM Swaziland - SWZ Sweden - SWE Switzerland - CHE Syrian Arab Republic - SYR Taiwan - TWN Tajikistan - TJK Tanzania - TZA Thailand - THA Togo - TGO Tokelau - TKL Tonga - TON Trinidad and Tobago - TTO Tunisia - TUN Turkey - TUR Turkmenistan - TKM Turks and Caicos Islands - TCA Tuvalu - TUV Uganda - UGA Ukraine - UKR United Arab Emirates - ARE United Kingdom - GBR United States - USA United States Minor Outlying Islands - UMI Uruguay - URY Uzbekistan - UZB Vanuatu - VUT Vatican City State - VAT Venezuela - VEN Viet Nam - VNM Virgin Islands (British) - VGB Virgin Islands (U.S.) - VIR Wallis and Futuna Islands - WLF Western Sahara - ESH Yemen - YEM Yugoslavia - YUG Zambia - ZMB Zimbabwe - ZWE
By
Jeremy Schnittman |
Published: Thursday, August 29, 2019
In this artist’s depiction of Cygnus X-1, a stellar-mass black hole strips gas from the surface of its companion star as they orbit each other. Since the 1970s, it has since become the strongest black hole candidate, with scientists at near certainty that it is one. Initially detected in X-ray, it has since been studied in various other spectra.
Albert Einstein developed his theory of gravity, known as general relativity, in 1915.
Karl Schwarzschild developed the idea for black holes from relativity’s equations in 1916, just a year after Einstein published his theory.
Cygnus X-1 (the brighter of the stars by the arrow) lies in a rich field near the plane of the Milky Way, and doesn’t look like much at visible wavelengths.
Cygnus X-1 first came to notice when astronomers found it to be an intense source of X-rays. In this view from the Chandra X-ray Observatory, the high-energy radiation is colored blue.
This simulation gives a realistic depiction of a black accretion disk, including the light-bending effects
of relativity.
JOIN THE DISCUSSION
Read and share your comments on this article
A chronicle of the first steps on the Moon , and what it took to get there.
With numb and trembling fingers, he opens the latest dispatches from home. One particularly bulky package attracts his attention. That night, throwing caution to the wind, he risks using an electric light to read the long and detailed report. Little does he know that it will prove to be arguably the most important work of creative genius of the 20th century.
The author of this pivotal document was a theoretical physicist named Albert Einstein. The recipient was his colleague Karl Schwarzschild, the director of the Astrophysical Observatory in Potsdam and an accomplished theorist and mathematician. Despite his astronomical career, Schwarzschild, then in his 40s, joined the war effort.
Just weeks before, Einstein had completed 10 long years of dedicated work, successfully expanding his special theory of relativity to include gravitational forces along with electricity and magnetism. In four landmark papers published in the Proceedings of the Prussian Academy of Sciences, Einstein laid out the mathematical foundation of the general theory of relativity, still considered one of the most beautiful and elegant scientific theories of all time.
Much like M. C. Escher’s famous picture of two hands sketching each other, the circular reasoning of Einstein’s field equations makes them both elegant, yet also notoriously difficult to solve. At the root of this difficulty is Einstein’s far more famous equation E=mc2, which states that energy and matter are interchangeable. Because gravity is a form of energy, it can behave like matter, creating yet more gravity. Mathematically speaking, general relativity is a nonlinear system. And nonlinear systems are really hard to solve.
It’s easy to imagine Einstein’s shock when, amid a dreadful war, Schwarzschild wrote back within a matter of days, describing the first known solution to Einstein’s field equations. Schwarzschild modestly writes, “As you see, the war treated me kindly enough, in spite of the heavy gunfire, to allow me to get away from it all and take this walk in the land of your ideas.” Einstein responds, “I have read your paper with the utmost interest. I had not expected that one could formulate the exact solution of the problem in such a simple way. I liked very much your mathematical treatment of the subject.”
Tragically, less than a year later, Schwarzschild succumbed to a skin disease contracted on the front, joining the millions of WWI fatalities due to disease. He left behind a solution that completely describes how space-time is warped outside a spherical object like a planet or star. One of the features of this mathematical solution is that for very compact, high-density stars, it becomes much harder to escape the gravitational field of the star. Eventually, there comes a point where every particle, even light, becomes gravitationally trapped. This point of no escape is called the event horizon. As one approaches the event horizon, time slows to a complete standstill.
Complicating the issue was the concurrent development of quantum mechanics, a new field almost entirely characterized by cases of nature behaving absurdly. Physicists working at the intersection of quantum mechanics and general relativity began to appreciate how both fields were critically important to understanding very massive and dense stars. But the bizarre nature of these new branches of physics strained even the most gifted intuition, so that even 50 years after Schwarzschild’s landmark paper, there was still no consensus on the existence of black holes.
Find the unseeable
One thing was clear: If black holes did exist, they were most likely formed by the collapse of massive stars, unable to support their own weight after running out of nuclear fuel. The question most astronomers were focused on was, “How do we find them?” After all, black holes give off no light of their own. Astronomy needs light, and to make light, you generally need matter — the hotter and brighter, the better.
Fortuitously, the late 1960s marked the dawn of X-ray astronomy with a series of sounding rockets and satellites that could get above Earth’s atmosphere, which otherwise blocks out all celestial X-rays.
During a short rocket flight in 1964, astronomers discovered one of the brightest X-ray sources in the sky, in the constellation Cygnus, dubbed Cygnus X-1 (Cyg X-1 for short). However, it didn’t coincide with any particularly bright optical or radio source, leaving its physical origin a mystery. When NASA’s Uhuru X-ray Explorer Satellite was launched in 1970, more detailed observations became possible, narrowing the uncertainty of its location. One of the first remarkable discoveries was Cyg X-1’s rapid variability, on timescales shorter than a second. This strongly suggested that the physical size of the X-ray-emitting region was quite compact, much smaller than a typical star. What could possibly pack so much power into such a small area?
As more sensitive X-ray telescopes were launched in subsequent years, the case only grew stronger. We have now seen X-ray variability from Cyg X-1 on timescales as short as a millisecond, confining the emission region to an extent of hundreds of kilometers, just a few times the size of the event horizon. By observing X-rays from black holes, we can directly probe the properties of space-time predicted by general relativity.
While stellar-mass black holes are some of the brightest X-ray sources in the sky, they are also some of the most fickle. In the 40-plus years since the discovery that Cyg X-1 is likely a black hole, only a few dozen more black hole candidates have been identified. Most of those have only been detectable during short, unpredictable outbursts lasting a month or so before they disappear again for decades. Compare that with their supermassive counterparts: The Sloan Digital Sky Survey alone has identified more than 100,000 quasars (the energetic centers of young, distant galaxies), each powered by an accreting supermassive black hole.
In addition to this most common “quiescent” behavior, astronomers have identified three other major states exhibited by stellar-mass black holes: hard, soft, and intermediate. These names describe the observable properties of the X-ray spectra in each state. We aren’t yet entirely certain what physical mechanisms drive these different behaviors, but they are likely tied to two things: how much gas the black hole is accreting, and how strong the magnetic fields embedded in the gas are.
In astronomical jargon, a “hard” spectrum means we see more high-energy X-rays than low-energy, and “soft” is the opposite. Of course, even “low-energy” is a relative term, as these photons come from an accretion disk that is at a temperature of millions of degrees, compared with the corona, which boasts a temperature in excess of 1 billion degrees!
The intermediate state shows evidence of a thin, cool accretion disk surrounded by a hot, diffuse corona like the surface of our own Sun. In this state, the high-energy X-rays coming from the corona shine down on the disk. Some of these X-rays get absorbed by the trace amounts of iron mixed in the disk’s gases. The iron then shines just like the fluorescent gas in a neon light, giving off more X-rays at very specific wavelengths. Because the gas in the disk is orbiting the black hole at nearly the speed of light, the X-rays coming from the disk experience extreme Doppler shifts, appearing to a distant observer at shorter wavelengths when the gas is moving toward the observer and longer wavelengths when moving away. By carefully measuring the wavelengths of the X-rays from an accreting black hole, we can measure how fast all the gas is orbiting around it.
Original spin
Considering the first solution to Einstein’s field equations took Schwarzschild less than a week to derive, it must have felt like an eternity to wait nearly a half-century before the next black hole solution was discovered by New Zealander Roy Kerr in 1963. (Another solution, the Reissner-Nordstrom black hole, was published almost immediately after Schwarzschild’s, but also is limited to spherically symmetric systems and mathematically almost identical.) Kerr made his formulation while at the University of Texas at Austin.
Evidence for this spin shows in how the black hole pulls everything around the horizon, essentially sweeping up space-time itself into a swirling vortex. This allows gas to move ever faster as it spirals closer and closer to the horizon, leading to more extreme Doppler shifts, and thus larger offsets in the X-ray spectra. In just the past few years since the launch of NASA’s NuSTAR X-ray telescope, we have been able to use these spectra to measure spins of multiple black holes with unprecedented accuracy. NuSTAR’s ability to see X-rays covering a much wider range of energies compared with previous missions also allows us to rule out other alternative models — like X-ray absorption by interstellar gas clouds — that had been proposed to explain the shape of the spectrum.
Measuring black hole spins not only teaches us about general relativity, but it also provides important insight into how massive stars evolve and collapse in supernovae. Because many of these binary systems are quite young (at least by cosmic standards — Cyg X-1 is “only” a few million years old), whatever spin we measure today is essentially the same spin that came from the original formation. From this point of view, they truly are “frozen stars,” retaining a near-perfect memory of their violent birth.
An outrageous legacy
General relativity is one of the few fields in modern physics where theory has driven experimentation for almost the entire century. Einstein had a unique talent for not only proposing brilliant and fruitful thought experiments, but also real experiments that could test his theories. Perhaps his most famous prediction was how the gravity of the Sun would deflect the light from distant stars, an effect confirmed with spectacular success in 1919 during a solar eclipse, propelling Einstein to international celebrity. More impressive still was the 40-plus years between Schwarzschild’s (unintentional) prediction of black holes and the discovery of Cyg X-1.
To borrow a phrase from theoretical physicist Kip Thorne, perhaps the most outrageous piece of Einstein’s legacy was his prediction of gravitational waves, made a century ago, and triumphantly confirmed just this year by the Laser Interferometer Gravitational-wave Observatory (LIGO). In addition to confirming the basic idea that the “fabric” of space-time is not just a metaphor but a tangible substance, the LIGO discovery also provided a new test of general relativity in the most extreme environment — just outside a black hole. There were some surprises in store, as well: the discovery of stellar-mass black holes 30 times the mass of the Sun, twice as big as any seen before. For the cherry on top, LIGO was even able to measure the spin of the final black hole at 70 percent of the maximum Kerr limit, arguably the most accurate and precise measure of spin to date.
The first puzzle came right on the heels of the first detection of Cyg X-1. In 1973, from the most basic laws of conservation of energy and angular momentum, Igor Novikov and Kip Thorne derived a brilliant and elegant description of how gas slowly spirals in toward a black hole, releasing its gravitational potential energy as heat and radiation at temperatures of millions of degrees.
There are only two problems with the Novikov-Thorne model: It doesn’t work in theory, and it doesn’t work in practice. It doesn’t work in theory because it doesn’t explain how exactly the gas loses angular momentum. It doesn’t work in practice because it doesn’t agree with observations of high-energy X-rays coming from billion-degree gas.
Hot ionized gas experiences almost no friction or viscosity, so it should simply go around and around on perfectly circular orbits forever, never getting any closer to the event horizon. Novikov and Thorne fully appreciated this problem, and they absorbed it into their theory with a simple fudge factor, leaving the details to later work. In the end, it took almost 20 years to find the answer. In 1991, Steve Balbus and John Hawley discovered a powerful instability that comes from the twisting and pulling of magnetic field lines embedded in an accretion disk. Ionized gas is an excellent electrical conductor, which means it also can generate powerful magnetic fields. These fields, in turn, can pull back on the gas, slowing it down and allowing it to spiral in toward the black hole.
By 2001, supercomputers had become powerful enough to adequately simulate the Balbus-Hawley instability in accretion disks around realistic black holes, fully confirming their predictions. It took yet another decade before the simulations were sophisticated enough to include the effects of radiation, and study the interplay between the disk and corona. In doing so, we have finally reached the point where, starting from the most fundamental laws of nature, we can explain how the high-energy X-rays, first seen in 1971, are actually generated around real black holes.
In exactly 100 years, black holes have progressed from being a mathematical curiosity, to the subject of p
Gagged Girl Sexy Ball
Handjob 1080
Black Hole