Heart
Health ResearchThe heart is a muscular organ in most animals, which pumps blood through the blood vesselsof the circulatory system. Blood provides the body with oxygen and nutrients, as well as assists in the removal of metabolic wastes. In humans, the heart is located between the lungs, in the middle compartment of the chest.
In humans, other mammals, and birds, the heart is divided into four chambers: upper left and right atria; and lower left and right ventricles. Commonly the right atrium and ventricle are referred together as the right heart and their left counterparts as the left heart. Fish, in contrast, have two chambers, an atrium and a ventricle, while reptiles have three chambers. In a healthy heart blood flows one way through the heart due to heart valves, which prevent backflow. The heart is enclosed in a protective sac, the pericardium, which also contains a small amount of fluid. The wall of the heart is made up of three layers: epicardium, myocardium, and endocardium.

The heart pumps blood with a rhythm determined by a group of pacemaking cells in the sinoatrial node. These generate a current that causes contraction of the heart, traveling through the atrioventricular node and along the conduction system of the heart. The heart receives blood low in oxygen from the systemic circulation, which enters the right atrium from the superior and inferior venae cavae and passes to the right ventricle. From here it is pumped into the pulmonary circulation, through the lungs where it receives oxygen and gives off carbon dioxide. Oxygenated blood then returns to the left atrium, passes through the left ventricle and is pumped out through the aorta to the systemic circulation−where the oxygen is used and metabolized to carbon dioxide. The heart beats at a resting rate close to 72 beats per minute. Exercise temporarily increases the rate, but lowers resting heart rate in the long term, and is good for heart health.
Cardiovascular diseases (CVD) are the most common cause of death globally as of 2008, accounting for 30% of deaths. Of these more than three quarters are a result of coronary artery disease and stroke. Risk factors include: smoking, being overweight, little exercise, high cholesterol, high blood pressure, and poorly controlled diabetes, among others. Cardiovascular diseases frequently do not have symptoms or may cause chest pain or shortness of breath. Diagnosis of heart disease is often done by the taking of a medical history, listening to the heart-sounds with a stethoscope, ECG, and ultrasound. Specialists who focus on diseases of the heart are called cardiologists, although many specialties of medicine may be involved in treatment.
Structure
Location and shape
The human heart is in the middle of the thorax, with its apex pointing to the left.
The human heart is situated in the middle mediastinum, at the level of thoracic vertebrae T5-T8. A double-membraned sac called the pericardium surrounds the heart and attaches to the mediastinum. The back surface of the heart lies near the vertebral column, and the front surface sits behind the sternum and rib cartilages. The upper part of the heart is the attachment point for several large blood vessels – the venae cavae, aorta and pulmonary trunk. The upper part of the heart is located at the level of the third costal cartilage. The lower tip of the heart, the apex, lies to the left of the sternum (8 to 9 cm from the midsternal line) between the junction of the fourth and fifth ribs near their articulation with the costal cartilages.
The largest part of the heart is usually slightly offset to the left side of the chest (though occasionally it may be offset to the right) and is felt to be on the left because the left heart is stronger and larger, since it pumps to all body parts. Because the heart is between the lungs, the left lung is smaller than the right lung and has a cardiac notch in its border to accommodate the heart. The heart is cone-shaped, with its base positioned upwards and tapering down to the apex. An adult heart has a mass of 250–350 grams (9–12 oz). The heart is typically the size of a fist: 12 cm (5 in) in length, 8 cm (3.5 in) wide, and 6 cm (2.5 in) in thickness. Well-trained athletes can have much larger hearts due to the effects of exercise on the heart muscle, similar to the response of skeletal muscle
Chambers
The heart has four chambers, two upper atria, the receiving chambers, and two lower ventricles, the discharging chambers. The atria open into the ventricles via the atrioventricular valves, present in the atrioventricular septum. This distinction is visible also on the surface of the heart as the coronary sulcus. There is an ear-shaped structure in the upper right atrium called the right atrial appendage, or auricle, and another in the upper left atrium, the left atrial appendage. The right atrium and the right ventricle together are sometimes referred to as the right heart. Similarly, the left atrium and the left ventricle together are sometimes referred to as the left heart. The ventricles are separated from each other by the interventricular septum, visible on the surface of the heart as the anterior longitudinal sulcus and the posterior interventricular sulcus.

Heart being dissected showing right and left ventricles, from above
The cardiac skeleton is made of dense connective tissue and this gives structure to the heart. It forms the atrioventricular septumwhich separates the atria from the ventricles, and the fibrous rings which serve as bases for the four heart valves. The cardiac skeleton also provides an important boundary in the heart's electrical conduction system since collagen cannot conduct electricity. The interatrial septum separates the atria and the interventricular septum separates the ventricles. The interventricular septum is much thicker than the interatrial septum, since the ventricles need to generate greater pressure when they contract.
Valves
The heart has four valves, which separate its chambers. One valve lies between each atrium and ventricle, and one valve rests at the exit of each ventricle.

With the atria and major vessels removed, all four valves are clearly visible.
The valves between the atria and ventricles are called the atrioventricular valves. Between the right atrium and the right ventricle is the tricuspid valve. The tricuspid valve has three cusps, which connect to chordae tendinae and three papillary muscles named the anterior, posterior, and septal muscles, after their relative positions. The mitral valve lies between the left atrium and left ventricle. It is also known as the bicuspid valve due to its having two cusps, an anterior and a posterior cusp. These cusps are also attached via chordae tendinae to two papillary muscles projecting from the ventricular wall.
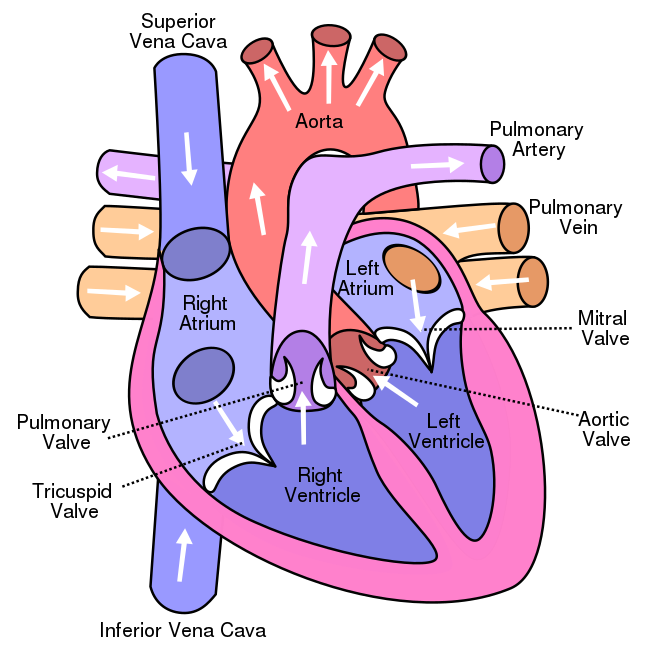
The heart, showing valves, arteries and veins. The white arrows show the normal direction of blood flow.
The papillary muscles extend from the walls of the heart to valves by cartilaginous connections called chordae tendinae. These muscles prevent the valves from falling too far back when they close. During the relaxation phase of the cardiac cycle, the papillary muscles are also relaxed and the tension on the chordae tendineae is slight. As the heart chambers contract, so do the papillary muscles. This creates tension on the chordae tendineae, helping to hold the cusps of the atrioventricular valves in place and preventing them from being blown back into the atria.
Two additional semilunar valves sit at the exit of each of the ventricles. The pulmonary valve is located at the base of the pulmonary artery. This has three cusps which are not attached to any papillary muscles. When the ventricle relaxes blood flows back into the ventricle from the artery and this flow of blood fills the pocket-like valve, pressing against the cusps which close to seal the valve. The semilunar aortic valve is at the base of the aorta and also is not attached to papillary muscles. This too has three cusps which close with the pressure of the blood flowing back from the aorta.

Frontal section showing papillary muscles attached to the tricuspid valve on the right and to the mitral valve on the left via chordae tendineae.
Right heart
The right heart consists of two chambers, the right atrium and the right ventricle, separated by a valve, the tricuspid valve.
The right atrium receives blood almost continuously from the body's two major veins, the superior and inferior venae cavae. A small amount of blood from the coronary circulation also drains into the right atrium via the coronary sinus, which is immediately above and to the middle of the opening of the inferior vena cava. In the wall of the right atrium is an oval-shaped depression known as the fossa ovalis, which is a remnant of an opening in the fetal heart known as the foramen ovale. Most of the internal surface of the right atrium is smooth, the depression of the fossa ovalis is medial, and the anterior surface has prominent ridges of pectinate muscles, which are also present in the right atrial appendage.
The right atrium is connected to the right ventricle by the tricuspid valve. The walls of the right ventricle are lined with trabeculae carneae, ridges of cardiac muscle covered by endocardium. In addition to these muscular ridges, a band of cardiac muscle, also covered by endocardium, known as the moderator band reinforces the thin walls of the right ventricle and plays a crucial role in cardiac conduction. It arises from the lower part of the interventricular septum and crosses the interior space of the right ventricle to connect with the inferior papillary muscle. The right ventricle tapers into the pulmonary trunk, into which it ejects blood when contracting. The pulmonary trunk branches into the left and right pulmonary arteries that carry the blood to each lung. The pulmonary valve lies between the right heart and the pulmonary trunk.
Left heart
The left heart has two chambers: the left atrium, and the left ventricle, separated by the mitral valve.
The left atrium receives oxygenated blood back from the lungs via one of the four pulmonary veins. The left atrium has an outpouching called the left atrial appendage. Like the right atrium, the left atrium is lined by pectinate muscles. The left atrium is connected to the left ventricle by the mitral valve.
The left ventricle is much thicker as compared with the right, due to the greater force needed to pump blood to the entire body. Like the right ventricle, the left also has trabeculae carneae, but there is no moderator band. The left ventricle pumps blood to the body through the aortic valve and into the aorta. Two small openings above the aortic valve carry blood to the heart itself, the left main coronary artery and the right coronary artery.
Heart wall
The heart wall is made up of three layers: the inner endocardium, middle myocardium and outer epicardium. These are surrounded by a double-membraned sac called the pericardium.

Layers of the heart wall, including visceral and parietal pericardium.
The innermost layer of the heart is called the endocardium. It is made up of a lining of simple squamous epithelium, and covers heart chambers and valves. It is continuous with the endotheliumof the veins and arteries of the heart, and is joined to the myocardium with a thin layer of connective tissue. The endocardium, by secreting endothelins, may also play a role in regulating the contraction of the myocardium.
The middle layer of the heart wall is the myocardium, which is the cardiac muscle – a layer of involuntary striated muscle tissue surrounded by a framework of collagen. The cardiac muscle pattern is elegant and complex, as the muscle cells swirl and spiral around the chambers of the heart, with the outer muscles forming a figure 8 pattern around the atria and around the bases of the great vessels and the inner muscles forming a figure 8 around the two ventricles and proceeding toward the apex. This complex swirling pattern allows the heart to pump blood more effectively.
There are two types of cells in cardiac muscle: muscle cells which have the ability to contract easily, and pacemaker cells of the conducting system. The muscle cells make up the bulk (99%) of cells in the atria and ventricles. These contractile cells are connected by intercalated discs which allow a rapid response to impulses of action potential from the pacemaker cells. The intercalated discs allow the cells to act as a syncytium and enable the contractions that pump blood through the heart and into the major arteries. The pacemaker cells make up 1% of cells and form the conduction system of the heart. They are generally much smaller than the contractile cells and have few myofibrils which gives them limited contractibility. Their function is similar in many respects to neurons. Cardiac muscle tissue has autorhythmicity, the unique ability to initiate a cardiac action potential at a fixed rate – spreading the impulse rapidly from cell to cell to trigger the contraction of the entire heart.

The swirling pattern of myocardium helps the heart pump effectively.
There are specific proteins expressed in cardiac muscle cells. These are mostly associated with muscle contraction, and bind with actin, myosin, tropomyosin, and troponin. They include MYH6, ACTC1, TNNI3, CDH2 and PKP2. Other proteins expressed are MYH7 and LDB3 that are also expressed in skeletal muscle.
Pericardium
The pericardium is the sack that surrounds the heart. The tough outer surface of the pericardium is called the fibrous membrane. This is lined by a double inner membrane called the serous membrane that produces pericardial fluid to lubricate the surface of the heart. The part of the serous membrane attached to the fibrous membrane is called the parietal pericardium, while the part of the serous membrane attached to the heart is known as the visceral pericardium. The pericardium is present in order to lubricate its movement against other structures within the chest, to keep the heart's position stabilised within the chest, and to protect the heart from infection.
Coronary circulation
Heart tissue, like all cells in the body, needs to be supplied with oxygen, nutrients and a way of removing metabolic wastes. This is achieved by the coronary circulation, which includes arteries, veins, and lymphatic vessels. Blood flow through the coronary vessels occurs in peaks and troughs relating to the heart muscle's relaxation or contraction.
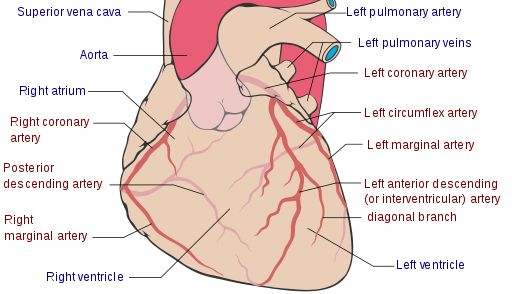
Arterial supply to the heart (red), with other areas labelled (blue).
Heart tissue receives blood from two arteries which arise just above the aortic valve. These are the left main coronary artery and the right coronary artery. The left main coronary artery splits shortly after leaving the aorta into two vessels, the left anterior descending and the left circumflex artery. The left anterior descending artery supplies heart tissue and the front, outer side, and the septum of the left ventricle. It does this by branching into smaller arteries – diagonal and septal branches. The left circumflex supplies the back and underneath of the left ventricle. The right coronary artery supplies the right atrium, right ventricle, and lower posterior sections of the left ventricle. The right coronary artery also supplies blood to the atrioventricular node (in about 90% of people) and the sinoatrial node (in about 60% of people). The right coronary artery runs in a groove at the back of the heart and the left anterior descending artery runs in a groove at the front. There is significant variation between people in the anatomy of the arteries that supply the heart. The arteries divide at their furtherst reaches into smaller branches that join together at the edges of each arterial distribution.
The coronary sinus is a large vein that drains into the right atrium, and receives most of the venous drainage of the heart. It receives blood from the great cardiac vein (receiving the left atrium and both ventricles), the posterior cardiac vein (draining the back of the left ventricle), the middle cardiac vein (draining the bottom of the left and right ventricles), and small cardiac veins. The anterior cardiac veins drain the front of the right ventricle and drain directly into the right atrium.
Small lymphatic networks called plexuses exist beneath each of the three layers of the heart. These networks collect into a main left and a main right trunk, which travel up the groove between the ventricles that exists on the heart's surface, receiving smaller vessels as they travel up. These vessels then travel into the atrioventricular groove, and receive a third vessel which drains the section of the left ventricle sitting on the diaphragm. The left vessel joins with this third vessel, and travels along the pulmonary artery and left atrium, ending in the inferior tracheobronchial node. The right vessel travels along the right atrium and the part of the right ventricle sitting on the diaphragm. It usually then travels in front of the ascending aorta and then ends in a brachiocephalic node.
Nerve supply
The heart receives nerve signals from the vagus nerve and from nerves arising from the sympathetic trunk. These nerves act to influence, but not control, the heart rate. Sympathetic nerves also influence the force of heart contraction. Signals that travel along these nerves arise from two paired cardiovascular centres in the medulla oblongata. The vagus nerve of the parasympathetic nervous system acts to decrease the heart rate, and nerves from the sympathetic trunk act to increase the heart rate. These nerves form a network of nerves that lies over the heart called the cardiac plexus.

Autonomic innervation of the heart.
The vagus nerve is a long, wandering nerve that emerges from the brainstem and provides parasympathetic stimulation to a large number of organs in the thorax and abdomen, including the heart. The nerves from the sympathetic trunk emerge through the T1-T4 thoracic ganglia and travel to both the sinoatrial and atrioventricular nodes, as well as to the atria and ventricles. The ventricles are more richly innervated by sympathetic fibers than parasympathetic fibers. Sympathetic stimulation causes the release of the neurotransmitter norepinephrine (also known as noradrenaline) at the neuromuscular junction of the cardiac nerves. This shortens the repolarization period, thus speeding the rate of depolarization and contraction, which results in an increased heart rate. It opens chemical or ligand-gated sodium and calcium ion channels, allowing an influx of positively charged ions. Norepinephrine binds to the beta–1 receptor.
Development
The heart is the first functional organ to develop and starts to beat and pump blood at about three weeks into embryogenesis. This early start is crucial for subsequent embryonic and prenatal development.

Development of the human heart during the first eight weeks (top) and the formation of the heart chambers (bottom). In this figure, the blue and red colors represent blood inflow and outflow (not venous and arterial blood). Initially, all venous blood flows from the tail/atria to the ventricles/head, a very different pattern from that of an adult.
The heart derives from splanchnopleuric mesenchyme in the neural plate which forms the cardiogenic region. Two endocardial tubes form here that fuse to form a primitive heart tube known as the tubular heart. Between the third and fourth week, the heart tube lengthens, and begins to fold to form an S-shape within the pericardium. This places the chambers and major vessels into the correct alignment for the developed heart. Further development will include the septa and valves formation and remodelling of the heart chambers. By the end of the fifth week the septa are complete and the heart valves are completed by the ninth week.
Before the fifth week, there is an opening in the fetal heart known as the foramen ovale. The foramen ovale allowed blood in the fetal heart to pass directly from the right atrium to the left atrium, allowing some blood to bypass the lungs. Within seconds after birth, a flap of tissue known as the septum primum that previously acted as a valve closes the foramen ovale and establishes the typical cardiac circulation pattern. A depression in the surface of the right atrium remains where the foramen ovale once walls, called the fossa ovalis.
The embryonic heart begins beating at around 22 days after conception (5 weeks after the last normal menstrual period, LMP). It starts to beat at a rate near to the mother's which is about 75–80 beats per minute (bpm). The embryonic heart rate then accelerates and reaches a peak rate of 165–185 bpm early in the early 7th week (early 9th week after the LMP). After 9 weeks (start of the fetal stage) it starts to decelerate, slowing to around 145 (±25) bpm at birth. There is no difference in female and male heart rates before birth.
Physiology
Blood flow
The heart functions as a pump in the circulatory system to provide a continuous flow of blood throughout the body. This circulation consists of the systemic circulation to and from the body and the pulmonary circulation to and from the lungs. Blood in the pulmonary circulation exchanges carbon dioxide for oxygen in the lungs through the process of respiration. The systemic circulation then transports oxygen to the body and returns carbon dioxide and relatively deoxygenated blood to the heart for transfer to the lungs.

Blood flow through the valves
The right heart collects deoxygenated blood from two large veins, the superior and inferior venae cavae. Blood collects in the right and left atrium continuously. The superior vena cava drains blood from above the diaphragm and empties into the upper back part of the right atrium. The inferior vena cava drains the blood from below the diaphragm and empties into the back part of the atrium below the opening for the superior vena cava. Immediately above and to the middle of the opening of the inferior vena cava is the opening of the thin-walled coronary sinus. Additionally, the coronary sinus returns deoxygenated blood from the myocardium to the right atrium. The blood collects in the right atrium. When the right atrium contracts, the blood is pumped through the tricuspid valve into the right ventricle. As the right ventricle contracts, the tricuspid valve closes and the blood is pumped into the pulmonary trunk through the pulmonary valve. The pulmonary trunk divides into pulmonary arteries and progressively smaller arteries throughout the lungs, until it reaches capillaries. As these pass by alveolicarbon dioxide is exchanged for oxygen. This happens through the passive process of diffusion.
In the left heart, oxygenated blood is returned to the left atrium via the pulmonary veins. It is then pumped into the left ventricle through the mitral valve and into the aorta through the aortic valve for systemic circulation. The aorta is a large artery that branches into many smaller arteries, arterioles, and ultimately capillaries. In the capillaries, oxygen and nutrients from blood are supplied to body cells for metabolism, and exchanged for carbon dioxide and waste products. Capillary blood, now deoxygenated, travels into venules and veins that ultimately collect in the superior and inferior vena cavae, and into the right heart.
Cardiac cycle
The cardiac cycle refers to the sequence of events in which the heart contracts and relaxes with every heartbeat. The period of time during which the ventricles contract, forcing blood out into the aorta and main pulmonary artery, is known as systole, while the period during which the ventricles relax and refill with blood is known as diastole. The atria and ventricles work in concert, so in systole when the ventricles are contracting, the atria are relaxed and collecting blood. When the ventricles are relaxed in diastole, the atria contract to pump blood to the ventricles. This coordination ensures blood is pumped efficiently to the body.

The cardiac cycle as correlated to the ECG
At the beginning of the cardiac cycle, the ventricles are relaxing. As they do so, they are filled by blood passing through the open mitral and tricuspid valves. After the ventricles have completed most of their filling, the atria contract, forcing further blood into the ventricles and priming the pump. Next, the ventricles start to contract. As the pressure rises within the cavities of the ventricles, the mitral and tricuspid valves are forced shut. As the pressure within the ventricles rises further, exceeding the pressure with the aorta and pulmonary arteries, the aortic and pulmonary valves open. Blood is ejected from the heart, causing the pressure within the ventricles to fall. Simultaneously, the atria refill as blood flows into the right atrium through the superior and inferior vena cavae, and into the left atrium through the pulmonary veins. Finally, when the pressure within the ventricles falls below the pressure within the aorta and pulmonary arteries, the aortic and pulmonary valves close. The ventricles start to relax, the mitral and tricuspid valves open, and the cycle begins again.
Cardiac output
Cardiac output (CO) is a measurement of the amount of blood pumped by each ventricle (stroke volume) in one minute. This is calculated by multiplying the stroke volume (SV) by the beats per minute of the heart rate (HR). So that: CO = SV x HR. The cardiac output is normalized to body size through body surface area and is called the cardiac index.

The x-axis reflects time with a recording of the heart sounds. The y-axis represents pressure.
The average cardiac output, using an average stroke volume of about 70mL, is 5.25 L/min, with a normal range of 4.0–8.0 L/min. The stroke volume is normally measured using an echocardiogram and can be influenced by the size of the heart, physical and mental condition of the individual, sex, contractility, duration of contraction, preload and afterload.
Preload refers to the filling pressure of the atria at the end of diastole, when they are at their fullest. A main factor is how long it takes the ventricles to fill—if the ventricles contract faster, then there is less time to fill and the preload will be less. Preload can also be affected by a person's blood volume. The force of each contraction of the heart muscle is proportional to the preload, described as the Frank-Starling mechanism. This states that the force of contraction is directly proportional to the initial length of muscle fiber, meaning a ventricle will contract more forcefully, the more it is stretched.
Afterload, or how much pressure the heart must generate to eject blood at systole, is influenced by vascular resistance. It can be influenced by narrowing of the heart valves (stenosis) or contraction or relaxation of the peripheral blood vessels.
The strength of heart muscle contractions controls the stroke volume. This can be influenced positively or negatively by agents termed inotropes. These agents can be a result of changes within the body, or be given as drugs as part of treatment for a medical disorder, or as a form of life support, particularly in intensive care units. Inotropes that increase the force of contraction are "positive" inotropes, and include sympathetic agents such as adrenaline, noradrenaline and dopamine. "Negative" inotropes decrease the force of contraction and include calcium channel blockers.
Electrical conduction
The normal rhythmical heart beat, called sinus rhythm, is established by the sinoatrial node, the heart's pacemaker. Here an electrical signal is created that travels through the heart, causing the heart muscle to contract.

Transmission of a cardiac action potentialthrough the heart's conduction system
The sinoatrial node is found in the upper part of the right atrium near to the junction with the superior vena cava. The electrical signal generated by the sinoatrial node travels through the right atrium in a radial way that is not completely understood. It travels to the left atrium via Bachmann's bundle, such that the muscles of the left and right atria contract together. The signal then travels to the atrioventricular node. This is found at the bottom of the right atrium in the atrioventricular septum—the boundary between the right atrium and the left ventricle. The septum is part of the cardiac skeleton, tissue within the heart that the electrical signal cannot pass through, which forces the signal to pass through the atrioventricular node only. The signal then travels along the bundle of His to left and right bundle branches through to the ventricles of the heart. In the ventricles the signal is carried by specialized tissue called the Purkinje fibers which then transmit the electric charge to the heart muscle.
Heart rate
The normal resting heart rate is called the sinus rhythm, created and sustained by the sinoatrial node, a group of pacemaking cells found in the wall of the right atrium. Cells in the sinoatrial node do this by creating an action potential. The cardiac action potential is created by the movement of specific electrolytes into and out of the pacemaker cells. The action potential then spreads to nearby cells.

Conduction system of the heart
When the sinoatrial cells are resting, they have a negative charge on their membranes. However a rapid influx of sodium ions causes the membrane's charge to become positive. This is called depolarisation and occurs spontaneously. Once the cell has a sufficiently high charge, the sodium channels close and calcium ions then begin to enter the cell, shortly after which potassium begins to leave it. All the ions travel through ion channels in the membrane of the sinoatrial cells. The potassium and calcium only start to move out of and into the cell once it has a sufficiently high charge, and so are called voltage-gated. Shortly after this, the calcium channels close and potassium channels open, allowing potassium to leave the cell. This causes the cell to have a negative resting charge and is called repolarization. When the membrane potential reaches approximately −60 mV, the potassium channels close and the process may begin again.
The ions move from areas where they are concentrated to where they are not. For this reason sodium moves into the cell from outside, and potassium moves from within the cell to outside the cell. Calcium also plays a critical role. Their influx through slow channels means that the sinoatrial cells have a prolonged "plateau" phase when they have a positive charge. A part of this is called the absolute refractory period. Calcium ions also combine with the regulatory protein troponin C in the troponin complex to enable contraction of the cardiac muscle, and separate from the protein to allow relaxation.
The adult resting heart rate ranges from 60 to 100 bpm. The resting heart rate of a newborn can be 129 beats per minute (bpm) and this gradually decreases until maturity. An athlete's heart rate can be lower than 60 bpm. During exercise the rate can be 150 bpm with maximum rates reaching from 200 to 220 bpm.

The prepotential is due to a slow influx of sodium ions until the threshold is reached followed by a rapid depolarization and repolarization. The prepotential accounts for the membrane reaching threshold and initiates the spontaneous depolarization and contraction of the cell; there is no resting potential.
Influences
The normal sinus rhythm of the heart, giving the resting heart rate, is influenced a number of factors. The cardiovascular centres in the brainstem that control the sympathetic and parasympathetic influences to the heart through the vagus nerve and sympathetic trunk. These cardiovascular centres receive input from a series of receptors including baroreceptors, sensing stretch the stretching of blood vessels and chemoreceptors, sensing the amount of oxygen and carbon dioxide in the blood and its pH. Through a series of reflexes these help regulate and sustain blood flow.
Baroreceptors are stretch receptors located in the aortic sinus, carotid bodies, the venae cavae, and other locations, including pulmonary vessels and the right side of the heart itself. Baroreceptors fire at a rate determined by how much they are stretched, which is influenced by blood pressure, level of physical activity, and the relative distribution of blood. With increased pressure and stretch, the rate of baroreceptor firing increases, and the cardiac centers decrease sympathetic stimulation and increase parasympathetic stimulation . As pressure and stretch decrease, the rate of baroreceptor firing decreases, and the cardiac centers increase sympathetic stimulation and decrease parasympathetic stimulation. There is a similar reflex, called the atrial reflex or Bainbridge reflex, associated with varying rates of blood flow to the atria. Increased venous return stretches the walls of the atria where specialized baroreceptors are located. However, as the atrial baroreceptors increase their rate of firing and as they stretch due to the increased blood pressure, the cardiac center responds by increasing sympathetic stimulation and inhibiting parasympathetic stimulation to increase heart rate. The opposite is also true. Chemoreceptors present in the carotid body or adjacent to the aorta in an aortic body respond to the blood's oxygen, carbon dioxide levels. Low oxygen or high carbon dioxide will stimulate firing of the receptors.
Exercise and fitness levels, age, body temperature, basal metabolic rate, and even a person's emotional state can all affect the heart rate. High levels of the hormones epinephrine, norepinephrine, and thyroid hormones can increase the heart rate. The levels of electrolytes including calcium, potassium, and sodium can also influence the speed and regularity of the heart rate; low blood oxygen, low blood pressure and dehydration may increase it.
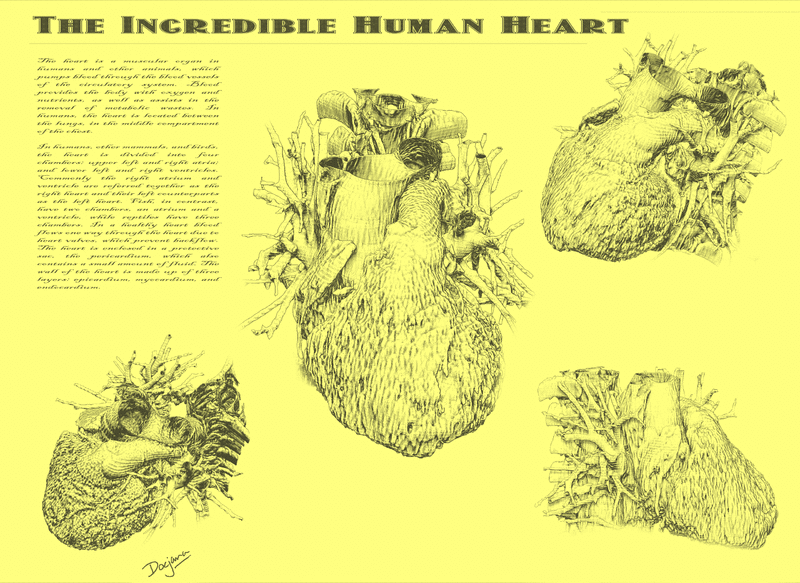